Obesity and heart failure with preserved ejection fraction: focus on new drugs and future direction in medical treatment
Article information
Abstract
Obesity is a major risk factor for heart failure with preserved ejection fraction (HFpEF) and contributes through multiple pathophysiological pathways, including systemic inflammation, neurohormonal activation, and mechanical inhibition. The treatment of obesity has shown significant potential for improving HFpEF outcomes. Sodium-glucose cotransporter 2 inhibitors have emerged as effective treatments for improving symptoms and quality of life in patients with HFpEF while aiding in weight control. Furthermore, a recent demonstration of the clinical benefits of glucagon-like peptide-1 receptor agonists (GLP-1 RAs) in HFpEF showed promising results in reducing weight loss, and improving symptoms and clinical outcomes. In this review article, we discuss the association between HFpEF and obesity, the emerging role of GLP-1 RAs, and future directions for medical therapies targeting obesity-associated HFpEF.
INTRODUCTION
Heart failure (HF) is a leading cause of morbidity and mortality worldwide. Despite advances in the treatment of HF, its prevalence continues to increase, and it is becoming more common in Korea [1–3]. HF is classified according to the ejection fraction (EF) of the left ventricle (LV) as HF with preserved EF (HFpEF), HF with mildly reduced EF (HFmrEF), and HF with reduced EF (HFrEF). Of these, HFpEF is estimated to account for more than 50% of all HF cases, and its prognosis is similar to that of HFrEF, making its diagnosis and treatment important [4]. HFpEF is difficult to diagnose owing to its highly heterogeneous phenotype and multiple causes [5–7]. Unlike HFrEF, which has a definite treatment option, there are no established medical therapies for HFpEF except for sodium-glucose cotransporter 2 (SGLT2) inhibitors and finerenone, which have been shown in recent clinical trials to be effective in preventing death and HF rehospitalization [8–10]. Instead, HFpEF requires emphasizing the management of each patient’s comorbidities [11–13].
Obesity is a major contributor to HFpEF, with evidence suggesting it is more closely associated with HFpEF than HFrEF. Up to 80% of patients with HFpEF in Western countries are reported to be overweight or obese [11]. Obesity is a chronic and complicated disease characterized by the accumulation of excess fat, which can compromise health. Obesity is defined as a state of increased fat accumulation, with a body mass index (BMI) of 30 or greater in the international definition and 25 or greater in Korea. The prevalence of obesity continues to increase worldwide, and the prevalence of obesity in Korea has also continued to rise, now standing at 38.4% [14]. The growing prevalence of obesity is also driving an increase in the prevalence of HF. BMI or waist circumference is a surrogate marker of fatness and is considered a strong predictor of HF, as several studies have shown a correlation between increased BMI or waist circumference and the development of HF [15–18]. Obesity is a risk factor for HFpEF on its own; it may also be a risk factor for several metabolic conditions such as diabetes, chronic kidney disease, and hypertension, which can lead to HFpEF [19]. However, the obesity paradox should not be overlooked. Although obesity is a significant risk factor for HF, patients with obesity previously diagnosed with HF often exhibit better prognoses and lower mortality rates than those without obesity, a phenomenon known as the obesity paradox [20]. This may be attributed to the limitations of BMI, which does not differentiate between fat and muscle mass [21]. Patients with obesity tend to maintain a relatively higher muscle mass, which can enhance cardiac output and systemic blood flow, thereby improving survival rates. In contrast, the absence of sufficient muscle mass may worsen HF outcomes, leading to a poorer prognosis in patients with a lower BMI.
In patients with obesity, weight loss alone has the advantage of ameliorating hemodynamic abnormalities via several mechanisms. Weight loss reduces the heart rate, mean arterial pressure, resting oxygen consumption, pulmonary capillary wedge pressure, and mean pulmonary arterial pressure. It also reduces systemic inflammation and mechanical inhibition, and alters tissue metabolism. It is associated with a lower risk of HF and improves both diastolic dysfunction and ventricular remodeling [22–26]. In recent years, glucagon-like peptide-1 receptor agonists (GLP-1 RAs) have demonstrated effective weight loss outcomes, and upcoming drugs such as GLP-1 RA combination agents have also raised expectations for effective weight loss [27,28]. In addition to effective weight loss, these drugs are expected to provide potential therapeutic benefits to patients with HFpEF, including improved symptoms and prognoses through hemodynamic improvements.
In this review article, we aimed to (1) confirm the association between HFpEF and obesity, (2) introduce the mechanisms and recent studies on GLP-1 RAs in HFpEF, and (3) suggest future therapeutic directions for obesity-associated HFpEF.
PATHOPHYSIOLOGY OF OBESITY IN HFpEF
Obesity contributes to HFpEF via several mechanisms (Fig. 1). In particular, obesity is strongly associated with atrial remodeling and pulmonary hypertension [29–31]. Obesity leads to increased fluid volume and diastolic dysfunction, which increase left atrial pressure, and sustained elevated pressure causes left atrial dilatation. This atrial remodeling leads to decreased atrial function and left ventricular diastolic dysfunction. It also affects the pulmonary veins and capillaries, and a sustained pressure load causes pulmonary hypertension owing to the dysfunction of pulmonary vascular endothelial cells and vascular smooth muscle thickening. These circumstances contribute to the development of HFpEF, leading to HF symptoms and a reduced quality of life.
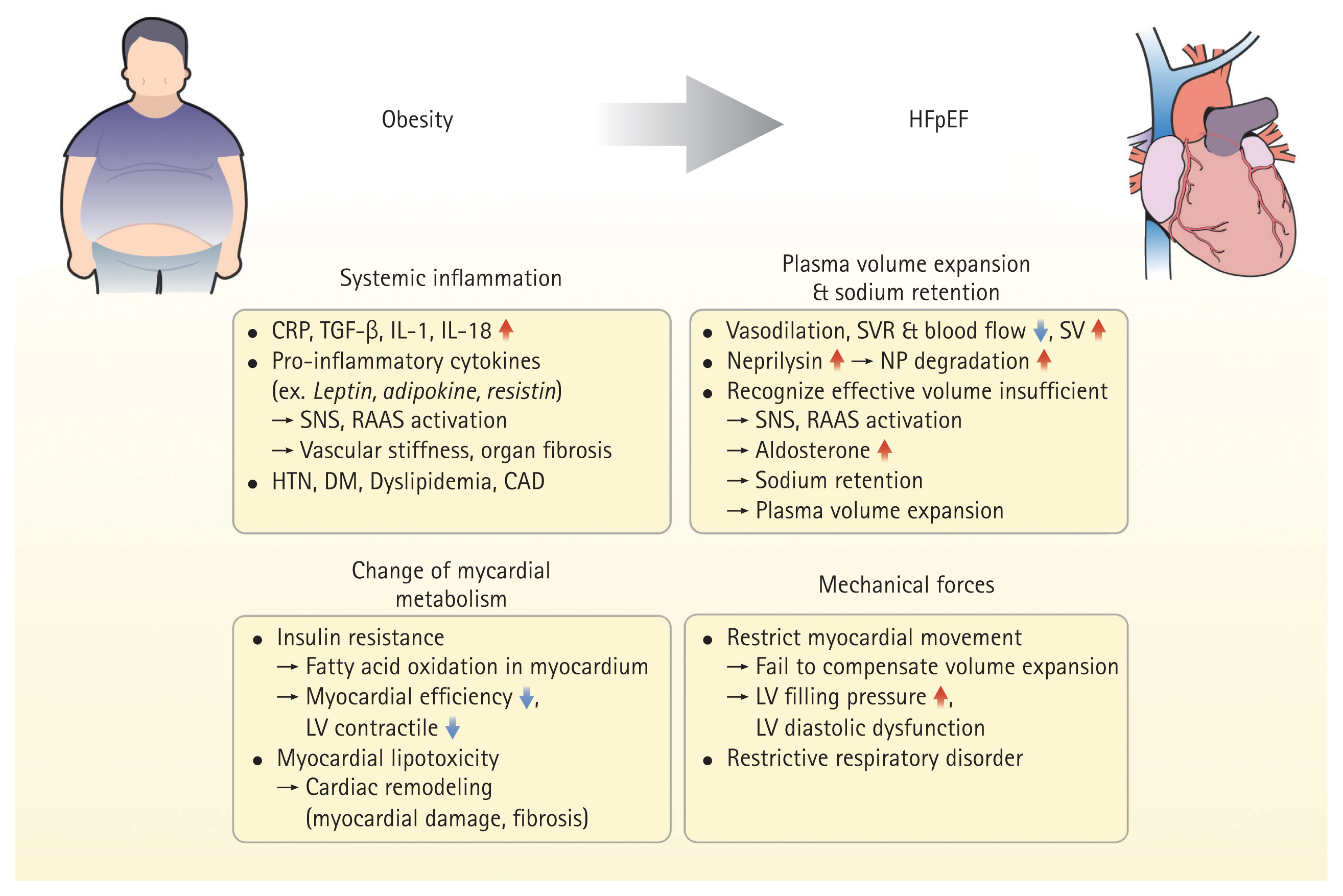
Pathophysiology of obesity and HFpEF. Obesity contributes to HFpEF via several mechanisms. HFpEF, heart failure with preserved ejection fraction; CRP, C-reactive protein; TGF, transforming growth factor; IL, interleukin; SNS, sympathetic nervous system; RAAS, renin-angiotensin-aldosterone system; HTN, hypertension; DM, diabetes mellitus; CAD, coronary artery disease; SVR, systemic vascular resistance; SV, stroke volume; NP, neprilysin; LV, left ventricle.
Physiologically, the adipose tissue exerts a protective effect on the heart by secreting adipokines that reduce cardiac hypertrophy, inflammation, and fibrosis. However, with the accumulation of excessive amounts of adipose tissue, adipokines switch to proinflammatory cytokines, causing damage to myocytes and systemic inflammation of the entire organ, which has adverse structural and functional effects on blood vessels and visceral organs [32–35]. Leptin is a major adipokine that regulates food intake and energy expenditure by acting on the sympathetic nervous system (SNS) and renin-angiotensin-aldosterone system (RAAS) to regulate neuroendocrine function and metabolism. However, in patients with obesity, the sensitivity of leptin receptors decreases, and leptin resistance increases, leading to a decrease in satiety signals and energy consumption, resulting in a relatively greater increase in fat mass [36]. In addition, the SNS and RAAS are constantly activated, resulting in a decrease in cardioprotective effects [37–39]. Other adipokines, such as resistin and adiponectin, induce cardiac hypertrophy and impair contractility with an increased N-terminal prohormone of brain natriuretic peptide (NT-proBNP), which adversely affects the risk of HF [23,40–42]. Consequently, in patients with obesity, the increased secretion of adipokines reduces their cardioprotective effects. In addition, elevated levels of C-reactive protein (CRP), a marker of inflammation, and increased secretion of transforming growth factor (TGF)-β, interleukin (IL)-1, and IL-18, which increase the risk of myocardial hypertrophy, fibrosis, and cardiac dysfunction, have been identified in obesity, leading to myocardial hypertrophy and fibrosis and eventually contributing to the development of HF [43,44]. Microvascular damage and fibrosis caused by inflammation leads to vascular stiffness, which may contribute to the development of HFpEF [45,46]. This inflammation can also affect visceral organs other than the heart, leading to conditions such as hypertension, diabetes, dyslipidemia, kidney disease, and coronary artery disease, which are common comorbidities in patients with HFpEF.
Obesity causes excessive vasodilation, decreased systemic vascular resistance and blood flow, and increased stroke volume without heart rate changes, resulting in high cardiac output [47]. Without adequate vascular resistance, sufficient tissue perfusion is not achieved, resulting in reduced oxygenation and activation of neurohormonal responses. Accordingly, the body recognizes that the effective blood volume is insufficient, and activates the RAAS and SNS. In addition, adipose tissue increases aldosterone levels by directly participating in aldosterone synthesis, and indirectly increases aldosterone secretion from the adrenal gland via leptin or catecholamines [38]. In obesity, neprilysin levels are increased in addition to leptin and aldosterone levels. This promotes natriuretic peptide degradation, and the overproduction of aldosterone and natriuretic peptide degradation lead to sodium retention, which affects plasma volume expansion and causes long-term changes in cardiac structure and function. HF exacerbates the overproduction of aldosterone and increases neprilysin levels, which in turn exacerbates HF and creates a vicious cycle [48].
In addition, insulin resistance develops in obesity [49]. The myocardium utilizes glucose and fatty acids as its main energy sources; however, in the presence of insulin resistance, changes in myocardial metabolism lead to increased fatty acid oxidation, which reduces myocardial efficiency, resulting in decreased (LV) contractility [50]. In addition, myocardial lipotoxicity, in which triglycerides or excess fatty acids accumulate in the myocardial tissue, affects cardiac remodeling, leading to myocardial damage and cardiac fibrosis [51].
Moreover, increased fat mass produces pathological mechanical forces that restrict myocardial movement, resulting in heart stiffness and difficulty in respiratory ventilation [52–54]. Epicardial fat normally supports and protects the myocardium via mechanical cushioning. However, in patients with obesity, large amounts of epicardial fat cause mechanical inhibition, making it difficult for the LV to compensate for the expanded volume. This increases ventricular filling pressure and causes left ventricular diastolic dysfunction. In addition, restrictive ventilation disorders due to chest wall restriction can also occur, resulting in resting and exercise-related respiratory disorders [55].
However, the association between obesity and HFrEF is still poorly understood, and obesity is not a universally observed cause, as in HFpEF. The impact of obesity on HFrEF has been largely attributed to its role as a risk factor for other cardiovascular (CV) diseases such as coronary artery disease or hypertension; however, recent studies have shown that in patients with non-ischemic cardiomyopathy, obesity may be a causative factor independent of idiopathic cardiomyopathy, suggesting that obesity may contribute to HFrEF by altering myocardial energetics or metabolism [56–58]. In addition, altered hemodynamics, mechanical effects, and systemic inflammation, which are the main mechanisms in the development of HFpEF, may ultimately act as major exacerbating factors of HFrEF [59]. The mechanisms through which obesity contributes to the pathogenesis of HFrEF require further investigation.
TREATMENTS FOR OBESITY IN HF
Current guidelines emphasize weight loss in patients with HF, particularly in those with a BMI of 35 or more [13,60,61]. The benefits of weight loss in obesity-related HF have been suggested by several studies, but data are limited, and clinical trials are lacking. Lifestyle modifications such as diet and exercise can reduce body weight, improve HF symptoms, and reduce the incidence of HF; however, weight loss through lifestyle modifications alone is limited [62]. There are some reports of favorable clinical outcomes in patients with HFpEF who have undergone bariatric surgery; however, there are no randomized clinical trials, and bariatric surgery is an invasive treatment. Therefore, anti-obesity drugs are becoming increasingly important and there is a need to study their effectiveness in the treatment of HF. In this context, a recent publication on the weight loss effects of GLP-1 RAs and their effectiveness in patients with HFpEF raises hope for the future role of anti-obesity drugs in HFpEF (Table 1).
Previous anti-obesity drugs and CV benefit
Anti-obesity drugs introduced before GLP-1 RAs were effective for weight loss; however, their benefits on CV outcomes remain unclear. Agents such as fenfluramine and sibutramine were removed because they increased the risk of significant valvular heart disease, myocardial disease, and stroke [63,64]. Phentermine is contraindicated in patients with CV risk factors because it increases the heart rate and blood pressure, which can increase the risk of arrhythmias, ischemic heart disease, and HF. Extended-release phentermine-topiramate reduces blood pressure without significantly changing heart rate, but its safety in CV disease has not been proven [65,66]. Tetrahydrolipstatin, also known by the trade name 'Orlistat', is a common anti-obesity drug that improves blood pressure, blood glucose, and hyperlipidemia; however, studies on patients with HF or CV risk factors are limited [67–69].
GLP-1 and GLP-1 RA
Food ingestion triggers the release of incretins from the gut, prolonging gastric emptying and promoting satiety, leading to reduced food intake and weight loss [70]. GLP-1 is a major incretin that promotes glucose-dependent insulin secretion from pancreatic beta cells and decreases glucose-dependent glucagon secretion from alpha cells, thereby increasing glucose uptake in peripheral tissues and inhibiting gluconeogenesis in the liver [70–72]. GLP-1 receptors are expressed in various organs and are associated with atrial and ventricular cardiomyopathies [73,74]. GLP-1 has multiple functions in addition to glycemic control and weight loss. Therefore, GLP-1 RA may act on multiple organs to reduce atherosclerosis-related events, exert anti-inflammatory effects, improve endothelial function, and modify risk factors through glycemic control (Fig. 2) [72,75].
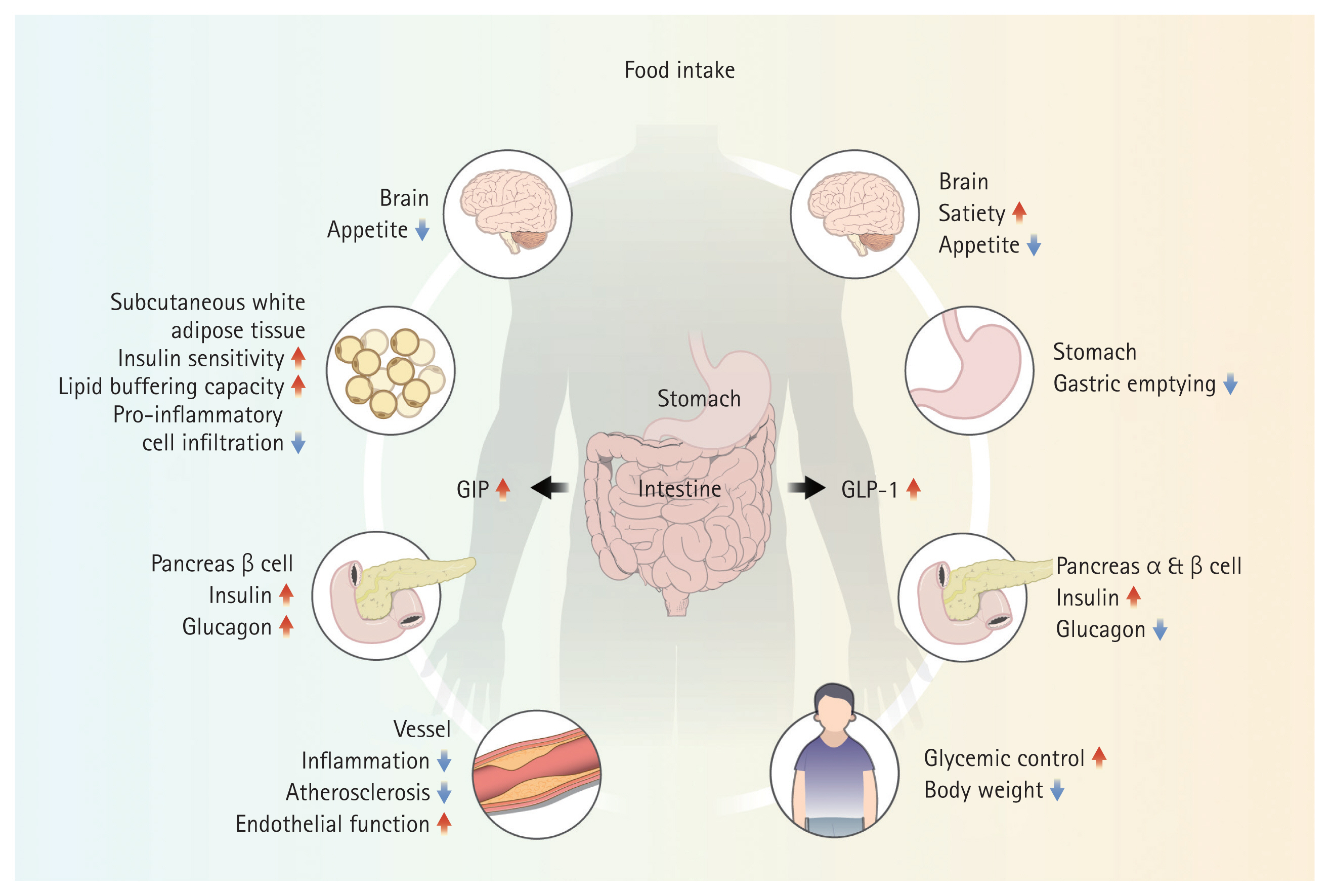
Mechanisms of GLP-1 and GIP. GLP-1 and GIP are secreted by the intestine following food intake. In the brain, it promotes satiety and loss of appetite; in the pancreas, GLP-1 increases insulin levels and decreases glucagon levels, whereas GIP increases both insulin and glucagon levels. Unlike GLP-1, GIP acts on subcutaneous white adipose tissue. GLP-1 and GIP are responsible for blood sugar control, weight loss, and anti-inflammatory, anti-atherosclerotic, and endothelial functions. Ultimately, these actions play a positive role in HFpEF. GLP-1, glucagon-like peptide-1; GIP, glucose-dependent insulin-tropic polypeptide; HFpEF, heart failure with preserved ejection fraction.
Current evidence of GLP-1 RAs for weight loss and CV disease
The main GLP-1 RAs, liraglutide and semaglutide, have been approved by the Food and Drug Administration for the treatment of obesity. Liraglutide resulted in 5.6 kg more weight loss than placebo, with 63.2% achieving a weight loss of at least 5% from baseline and 33.1% achieving a weight loss of at least 10% [76]. It has also been shown to reduce major adverse cardiovascular events (MACE) by 13% in patients with diabetes [77]. The STEP (Semaglutide Treatment Effect in People with Obesity) program, which explored the weight loss effects of semaglutide, found that a weekly dose of 2.4 mg plus lifestyle modification resulted in a weight loss of approximately 10% from baseline, regardless of diabetes status or race [78]. Semaglutide has also been shown to improve CV outcomes, with the SELECT (Semaglutide Effects on Cardiovascular Outcomes in People with Overweight or Obesity) trial confirming that semaglutide reduced the composite risk of death from CV causes, non-fatal myocardial infarction, or non-fatal stroke in patients with obesity by 20% compared to controls [79,80].
Current evidence of GLP-1 RAs for HFrEF
Most clinical trials for GLP-1 RAs did not consider HF hospitalization as a main outcome, so HF-related outcomes were of little interest, but one meta-analysis reported that GLP-1 RA could reduce HF admission by approximately 11%, which led us to hypothesize that GLP-1 RAs might be beneficial for HF-related events [81,82]. However, in the LIVE (Effect of Liraglutide on Left Ventricular Function in Stable Chronic Heart Failure Patients) study, which investigated the effect of GLP-1 RAs on LV systolic function in patients with EF ≤ 45%, liraglutide did not demonstrate improvement in LV systolic function, but rather increased heart rate and cardiac adverse events [83,84]. In addition, in patients with HFrEF with EF ≤ 40%, liraglutide did not reduce the death or HF hospitalization rates [85]. In contrast, a recent pre-specified analysis of the SELECT trial for semaglutide and CV outcomes in patients with obesity and prevalent HF confirmed the efficacy of semaglutide in patients with HFrEF [86]. This study clearly demonstrated a reduction in MACE, CV death, and HF hospitalization in patients with HF, and the risk reduction was more pronounced in patients with HFrEF. Therefore, the effectiveness of GLP-1 RAs in HFrEF requires further investigation.
Current evidence of GLP-1 RAs for HFpEF
In non-obese patients with HFpEF, weight loss is associated with increased all-cause mortality or rehospitalization rates and worsening physical function or nutritional status. However, in patients with obesity and HFpEF, weight loss is associated with improved peak oxygen consumption, exercise capacity, and symptoms, and reduced HF events, rehospitalization rates, and ventricular remodeling. Recent studies have shown a clear clinical benefit of GLP-1 RA in HFpEF, in contrast to the findings in HFrEF. The STEP-HFpEF trial administered 2.4 mg of semaglutide weekly to patients with obesity and HFpEF to determine the effectiveness of a composite of change in Kansas City Cardiomyopathy Questionnaire (KCCQ) score, change in body weight, change in 6-minute walk test, and composite outcome of death and HF hospitalization or urgent visit [87]. Over 52 weeks, the placebo group improved to 8.7 points and the semaglutide group to 16.6 points of KCCQ score, a difference of 7.8 (p < 0.001). Weight loss was 13.3% in the semaglutide group, 10.7% greater than that in the placebo group. The 6-minute walk test showed a significant difference of 21.5 meters in the semaglutide group and 1.2 meters in the placebo group. The overall outcome was also better in the semaglutide group with a win ratio of 1.72 (95% confidence interval [CI], 1.37–2.15; p < 0.001), and CRP, a marker of inflammation, changed by −43.5% from the baseline in the semaglutide group, compared to +7.3% from the baseline in the placebo group. Consequently, semaglutide not only provided effective weight loss in patients with obesity and HFpEF but also improved symptoms, quality of life, exercise tolerance, and clinical outcomes. Similarly, the STEP-HFpEF DM (Semaglutide in Patients with Obesity-Related Heart Failure and Type 2 Diabetes) trial in patients with obesity, HFpEF, and diabetes showed similar results to STEP-HFpEF over a one-year follow-up period [88]. Semaglutide has been shown to improve symptoms associated with congestion and volume overload, while reducing or maintaining doses in patients taking loop diuretics, and a reduction in NT-proBNP has been observed [79,89]. The reduction in NT-proBNP levels was more pronounced in lean patients than in patients with obesity, suggesting that NT-proBNP may have effects other than weight loss [90]. In a pooled analysis of clinical trials for semaglutide, semaglutide reduced the composite risk of CV death and HF events (hazard ratio [HR], 0.69; 95% CI, 0.53–0.89; p = 0.0045) and also significantly reduced the risk of worsening HF (HR, 0.59; 95% CI, 0.41–0.82; p = 0.0019) (Table 2) [91]. Recently, the PRAISE HFpEF DM study, a multicenter observational cohort study conducted in the United States, was presented [92]. This study evaluated the impact of GLP-1 RAs on clinical outcomes, specifically, a composite of HF hospitalization and all-cause mortality—in 1,024 patients with obesity and HFpEF compared with 796 controls receiving dipeptidyl peptidase inhibitors or sulfonylureas. The results demonstrated a 20% reduction in the risk of the primary outcomes in the GLP-1 RA group (HR, 0.80; 95% CI, 0.68–0.99).
The mechanism of action of GLP-1 RAs in HFpEF remains uncertain; however, greater weight loss has been shown to be associated with greater improvement in HF-related symptoms [93]. However, patients with more severe forms of HF, such as those with higher NT-proBNP levels, higher New York Heart Association class, atrial fibrillation, or those taking diuretics, showed similar weight loss but greater improvements in HF-related symptoms [94–96]. In addition, inflammatory markers were reduced independently of weight loss [87], suggesting that the effects of GLP-1 RAs on HF are likely due to the direct action on HF pathophysiology, in addition to the hemodynamic effects through weight loss. Therefore, in addition to the efficacy of GLP-1 RAs in improving clinical outcomes and symptoms in patients with HFpEF, further studies should be conducted to identify the underlying mechanisms.
SGLT2 inhibitors, which have recently been strongly recommended for the treatment of HFpEF, are also effective against obesity-related HFpEF. SGLT2 inhibitors are involved in regulating sodium retention and systemic inflammation and are also effective in weight loss through glucosuria. Dapagliflozin resulted in a weight loss of 3.5 kg compared to placebo and was effective in controlling symptoms, resulting in a 3.7 point increase in KCCQ score. Empagliflozin similarly reduced body weight by 1.28 kg (Table 3). Some studies have shown that a combination of SGLT2 inhibitors and GLP-1 RAs may provide CV and renal benefits to patients with HFpEF and diabetes. One study reported that a combination of SGLT2 inhibitors and GLP-1 RAs improved clinical outcomes, including hospitalization for HF [97]. Another study demonstrated that this combination therapy significantly reduced the risk of HF-related events compared with monotherapy [98]. These findings suggest that the combined use of SGLT2 inhibitors and GLP-1 RAs may further reduce the risk of HF progression and hospitalization in patients with HFpEF and diabetes. Angiotensin receptor neprilysin inhibitor is also thought to be effective in patients with obesity with increased neprilysin levels; however, studies in patients with HFpEF have not shown meaningful clinical outcomes compared with valsartan, and excluded patients with a BMI > 40 kg/m2, making its effectiveness in obesity-related HFpEF unclear [99,100]. Given the mechanisms involved in sodium retention and high aldosterone levels, mineralocorticoid antagonists (MRAs) may also be effective against obesity-associated HFpEF. Recently, a non-steroidal MRA, finerenone, has shown positive results in patients with HFpEF [10], and although it has not yet been studied in patients with obesity, a post-hoc analysis of the TOPCAT trial, which was based on another MRA, spironolactone, showed that spironolactone improved clinical outcomes in patients with obesity, suggesting that finerenone may be a promising treatment for obesity-related HFpEF [101,102]. However, finerenone is known to cause only minor weight loss [103]. In this respect, GLP-1 RAs are novel drugs that can effectively induce weight loss in patients with obesity-related HFpEF and improve the clinical outcomes, symptoms, and quality of life of patients with HFpEF better than other HFpEF treatments.
Future anti-obesity drug candidates for HFpEF
Dual gastric inhibitory polypeptide/GLP-1RA
Glucose-dependent insulin-tropic polypeptide (GIP), also known as gastric inhibitory polypeptide is another incretin with functions similar to those of GLP-1. It suppresses appetite, inhibits fat accumulation, reduces energy intake and increases energy expenditure. However, unlike GLP-1, GIP acts on subcutaneous white adipose tissue to increase insulin sensitivity and has additional roles, such as increased lipid buffering capacity, increased blood flow and storage capacity, and infiltration of proinflammatory immune cells [104]. Therefore, the combination of GLP-1 and GIP has a superior weight loss effect compared with other anti-obesity drugs, and this effect increases in a dose-dependent manner. Tirzepatide is a representative GLP-1/GIP combination drug. The SURMOUNT-1 (Tirzepatide Once Weekly for the Treatment of Obesity) trial showed a dose-dependent weight loss of 15–25% from baseline at doses of 5, 10, and 15 mg [105]. A clinical trial on the CV outcomes of tirzepatide in patients with HFpEF and obesity was recently reported. The SUMMIT (Study of Tirzepatide in Participants with Heart Failure with Preserved Ejection Fraction and Obesity) trial evaluated the efficacy and safety of once-weekly doses of tirzepatide on weight loss, HF symptoms, and clinical outcomes, including all-cause mortality and HF events, in patients with HFpEF with and without diabetes [106]. Similar to semaglutide, tirzepatide lowered the risk of death from worsening of HF or CV causes in patients with HFpEF and obesity. Another ongoing clinical trial of tirzepatide, SURMOUNT MMO (Effect of Tirzepatide on the Reduction of Morbidity and Mortality in Adults with Obesity, NCT05556512), aims to investigate CV outcomes in patients who are overweight or with obesity with a high risk of CV complications, including both HFrEF and HFpEF, and is expected to determine weight loss and clinical outcomes such as mortality and HF hospitalization in patients with high-risk HF.
Combination of GLP-1 RAs and glucagon receptor agonist
Glucagon receptor agonists reduce glucagon release, improve blood sugar levels, reduce food intake, and increase energy expenditure [107]. Survodutide is a dual GLP-1/glucagon receptor agonist. Phase 2 clinical trial results demonstrated a weight loss effect of 14.9% compared with baseline at 46 weeks, and trials on CV results are currently in progress [108]. Pemvidutide is another dual GLP-1/glucagon receptor agonist; however, its clinical data have not yet been reported. Retatrutide is a triple GIP/GLP-1/glucagon receptor agonist [109]. Phase 2 trial results showed that retatrutide had a dose-dependent weight loss effect, and in patients administered 12 mg of retatrutide, an average weight loss of 24% was observed at 48 weeks compared to baseline [110].
Oral GLP-1 RA
Orforglipron is an oral GLP-1 RA that showed a 14.7% change in body weight compared to baseline at 36 weeks at a 45 mg dose [111]. Studies are currently underway to determine its benefits on CV risk compared to insulin.
Combination of GLP-1 RA and amylin antagonist
Cagrilintide/semaglutide is a combination of a GLP-1 RA and a long-acting amylin analog. Amylin promotes delayed gastric emptying and increased satiety. In patients with overweight and DM, a 15.6% weight loss from baseline was demonstrated at week 32. A phase 3 clinical trial is currently underway to validate MACE and HF outcomes [112].
Confirmation of the effectiveness and safety of these drugs remains challenging. Although the pooled analysis of semaglutide identified the clinical outcomes of HF events and CV mortality, these were not primary outcomes, and evidence of substantial clinical outcome improvement is still needed. However, semaglutide, a GLP-1 RA, has demonstrated symptomatic improvement in HFpEF, and the GIP/GLP-1 dual agonist is expected to have positive effects on HFpEF. Therefore, anti-obesity drugs based on GLP-1 RA are expected to be potential candidates for the future treatment of HFpEF. Further randomized controlled trials are necessary to confirm these findings. In addition to GLP-1 RA-based therapies, recent studies have investigated drugs that target inflammatory pathways in patients with HFpEF (NCT05636176). Ziltivekimab, an IL-6 inhibitor, has the potential to reduce the systemic inflammation that contributes to myocardial stiffness, diastolic dysfunction, and abnormal remodeling observed in HFpEF. Therefore, it is a promising therapeutic candidate for patients with obesity and HFpEF. Further research is needed to validate its efficacy and safety in this population, paving the way for its potential role as an anti-obesity drug candidate for the complex treatment of HFpEF.
CONCLUSION
Obesity is a major risk factor for HF, particularly HFpEF. Patients with HFpEF and obesity experience more severe HF symptoms, lower quality of life, and more frequent admissions for HF aggravation. With the increasing incidence and prevalence of obesity and HFpEF, there is a growing demand for effective strategies for treating patients with obesity and HF. In addition to weight loss, GLP-1 RAs have been shown to improve symptoms, increase quality of life, and decrease clinical outcomes in patients with HFpEF. Although the mechanism of action of GLP-1 RAs in patients with obesity and HFpEF is not yet fully understood, the obvious benefits of GLP-1 RAs in this population have been demonstrated. Therefore, continued research on the role of GLP-1 RA-based anti-obesity drugs is expected to present a new treatment paradigm for HFpEF.
Notes
CRedit authorship contributions
Se-Eun Kim: writing - original draft, writing - review & editing, visualization; Byung-Su Yoo: conceptualization, writing - review & editing, supervision, funding acquisition
Conflicts of interest
The authors disclose no conflicts.
Funding
This work was supported by the Korea Disease Control and Prevention Agency (Nos. 2022-ER0908-00, 2022-ER0908-01, 2022-ER0908-02, 2023-ER0806-01).