Atorvastatin inhibits osteoclast differentiation by suppressing NF-κB and MAPK signaling during IL-1β-induced osteoclastogenesis
Article information
Abstract
Background/Aims
To define the effect of statins on interleukin 1β (IL-1β)-induced osteoclastogenesis and elucidate the underlying mechanisms.
Methods
Bone marrow cells were obtained from 5-week-old male ICR (Institute for Cancer Research) mice, and they were cultured to differentiate them into osteoclasts with macrophage colony-stimulating factor and the receptor activator of nuclear factor (NF)-κB ligand in the presence or absence of IL-1β or atorvastatin. The formation of osteoclasts was evaluated by tartrate-resistant acid phosphatase (TRAP) staining and resorption pit assay with dentine slice. The molecular mechanisms of the effects of atorvastatin on osteoclastogenesis were investigated using reverse transcription polymerase chain reaction and immunoblotting for osteoclast specific molecules.
Results
Atorvastatin significantly reduced the number of TRAP-positive multinucleated cells as well as the bone resorption area. Atorvastatin also downregulated the expression of the NF of activated T-cell c1 messenger RNA and inhibited the expression of osteoclast-specific genes. A possible underlying mechanism may be that atorvastatin suppresses the degradation of the inhibitors of NF-κB and blocks the activation of the c-Jun N-terminal kinase, extracellular signal-regulated kinase, and p38; thus, implicating the NF-κB and mitogen-activated protein kinases pathway in this process.
Conclusions
Atorvastatin is a strong inhibitor of inflammation-induced osteoclastogenesis in inflammatory joint diseases.
INTRODUCTION
Bone erosion is a central feature of rheumatoid arthritis (RA), and it is associated with disease severity and poor functional outcome. Bone loss results from an imbalance in which bone resorption by osteoclasts is favored over bone formation by osteoblasts [1]. A role for osteoclasts in bone erosion in RA was identified in multinucleated cells at sites of erosion in subchondral bone and at the pannus/bone interface in tissue samples from patients with RA [2].
The pathogenesis of bone erosion and the role of osteoclasts in RA were greatly augmented by the discovery of a cytokine system of osteoclast differentiation and activation. Osteotropic agents such as interleukin 1 (IL-1), IL-6, IL-11, IL-15, IL-17, tumor necrosis factor α (TNF-α), prostaglandin E2, and the parathyroid hormone induce bone loss by increasing osteoclast forma tion [3,4]. A cell-surface molecule, the receptor activator of nuclear factor (NF)-κB ligand (RANKL), recruits TNF receptor-associated factor (TRAF) proteins by binding to its receptor, the receptor activator of NF-κB (RANK), and it has been found to be a key factor in the stimulation of osteoclast formation [5,6]. TRAFs activate various signaling pathways, including c-Src, PI3-kinase/Akr, and mitogen-activated protein kinase (MAPK) [7]. RANKL activates numerous transcription factors, including c-Fos, NF of activated T-cell (NFAT) c1, PU1, and microphthalmia-associated transcription factors, as these are essential to osteoclast differentiation [8]. c-Fos is responsible for the expression of NF of activated T-cells (NFATc1) during RANKL-mediated osteoclast differentiation [9,10].
Atorvastatin is a 3-hydroxy-3-methylglutaryl coenzyme A (HMG-CoA) reductase inhibitor widely used as a lipid-lowering agent. Inhibition of the enzyme results in reduced conversion of HMG-CoA to mevalonate and subsequent isoprenoid precursors, and it mainly reduces the cholesterol level in liver cells [11]. Reduced cholesterol availability leads to increased liver low density lipoprotein (LDL) receptor synthesis and increased removal of LDL from the bloodstream [12]. Several studies have shown that statins such as atorvastatin may also exert an immunomodulatory effect by the inhibition of proinflammatory cytokine production [13], as well as an inhibitory effect on T-cell recruitment and T-cell stimulation [14,15]. Furthermore, atorvastatin may potentially have beneficial effects on bone metabolism in patients with hypercholesterolemia, mostly by reducing bone resorption rather than by stimulating bone formation [16].
Herein, we showed that the inhibitory mechanism of atorvastatin on IL-1β-induced, RANKL-mediated osteoclast differentiation via the MAPK/NF-κB pathway.
METHODS
Reagents and antibodies
Recombinant human IL-1β was purchased from R&D System (Minneapolis, MN, USA). Atorvastatin was obtained from Axon MedChem (Groningen, the Netherland). Human soluble RANKL and the macrophage-colony stimulating factor (M-CSF) were obtained from PeproTech (Rocky Hill, NJ, USA). Monoclonal antibodies (Abs) against extracellular signal-regulated kinase (ERK) 1/2, phosphate (p)-ERK, c-Jun N-terminal kinases (JNK), p-JNK, p38, p-p38, NF-κB (p65), inhibitor of NF-κBα (IκBα), and β-actin were purchased from Cell Signaling Technology (Danvers, MA, USA). Abs against c-Fos, NFATc1, and tartrate-resistant acid phosphatase (TRAP) were purchased from Santa Cruz Biotechnology (Dallas, TX, USA). The goat anti-rabbit immunoglobulin G (IgG, ENZO Life Sciences, Farmingdale, NY, USA) and rabbit anti-goat horseradish peroxidase (HRP)-conjugate were purchased from Bethyl Laboratories (Montgomery, TX, USA).
Osteoclast differentiation
Bone marrow cells (BMCs) were collected from the tibias and femurs with α-minimum essential media (α-MEM, WelGENE, Gyeongsan, Korea) containing antibiotics (Sigma-Aldrich, St. Louis, MO, USA) of 5-week-old male Institute for Cancer Research (ICR) mice. The animal experiments were approved by the Institutional Animal Care and Use Committee of Chonbuk National University (CNU), and they were conducted in accordance with the guidelines of CNU. The BMCs were cultured in α-MEM containing 10% fetal bovine serum (FBS), antibiotics, and M-CSF (10 ng/mL), and then they were cultured for 1 day. Nonadherent cells were plated on 100mm petri dishes and were then cultured for 3 days in the presence of M-CSF (30 ng/mL). Adherent cells were used as bone marrow-derived macrophages (BMMs). The BMMs were cultured for 3 days with M-CSF (30 ng/mL) and RANKL (100 ng/mL) to induce pre-fusion osteoclasts. To differentiate osteoclasts from the BMMs, they were cultured for 4 days with M-CSF (30 ng/mL) and RANKL (50 ng/mL) in the presence or absence of IL-1β (10 ng/mL) or atorvastatin (0.5 μM).
RNA isolation and reverse transcription-polymerase chain reaction
Total RNA was isolated with TRIzol reagent (Invitrogen, Carlsbad, CA, USA) according to the manufacturer’s recommended protocol. The complement (c)-DNA was synthesized using a Maxime RT Premix Kit (iNtRON Biotechnology, Seongnam, Korea), and then cDNA was amplified using a Maxime polymerase chain reaction (PCR) Premix Kit (iNtRON Biotechnology). The primers used in the PCR were as follows: NFATc1 forward, 5′-tgtgcaagccaaattccctg-3′, reverse, 5′-atacacccccagaccgcatc-3′; c-Fos forward, 5′-atcggaggagggagctgaca-3′, reverse, 5′-ggaaccggacaggtccacat-3′; TRAP forward, 5′-gtgctggctggaaaccatga-3′, reverse, 5′-cccactcagcacatagccca-3′; E-cadherin forward, 5′-aaatgatgtggctcccaccc-3′, reverse, 5′-catctcccatggtgccacac-3′; cathepsin K forward, 5′-cagagtgggaaggcagggtc-3′, reverse, 5′-gctttctcgttccccacagg-3′; osteoclast-associated receptor (OSCAR) forward, 5′-ctttttctttctggccgcgt-3′, reverse, 5′-catggtggctcacacccatc-3′; dendritic cell-specific transmembrane protein (DC-STAMP) forward, 5′-gcaaggaacccaaggagtcg-3′, reverse, 5′-cagttggcccagaaagaggg-3′; c-Src forward, 5′-ctggttccacggcaagatca-3′, reverse, 5′-ttcatgttcagtgcccagcc-3′; and glyceraldehyde 3-phosphate dehydrogenase (GAPDH) forward, 5′-tcatcatctccgccccttct-3′, reverse, 5′-agcttcccgttcagctctgg-3′. PCR products were electrophoresed on 1% agarose gel stained with ethidium bromide. Densitometric analysis was performed using FusionCapt Advance software (Vilber Lourmat, Eberhardzell, Germany).
Cell viability analysis
Cell viability was determined using a CCK-8 kit (Dojindo Laboratories, Kumamoto, Japan) according to the manufacturer’s instructions. Briefly, 2-(2-methoxy-4-nitrophenyl)-3-(4-nitrophenyl)-5-(2,4-disulfophenyl)-2Htetrazolium (CCK-8) was reduced by dehydrogenases in cells to yield an orange-colored product (formazan) [17]. The amount of formazan dye generated by dehydrogenases in the cells was directly proportional to the number of living cells. The BMMs were plated on 96-well plates with α-MEM containing 10% FBS and antibiotics with M-CSF (30 ng/mL) and RANKL (100 ng/mL) for 3 days. The cells were washed with complete α-MEM media and were then incubated for 24 hours with IL-1β (10 ng/mL) or atorvastatin (0.5 μM) in the presence of M-CSF (30 ng/mL) and RANKL (50 ng/mL). CCK-8 (10 μL/well) was added to each well of the plates, and the cells were incubated for 3 hours. The absorbance was measured at 450 nm using a microplate reader.
Western blot analysis
Cultured cells were lysed in the lysis buffer (50 mM Tris-HCl, 150 mM NACl, 5 mM ethylenediaminetetraacetic acid, 1% Triton X-100, 1 mM sodium fluoride, 1 mM sodium vanadate, and 1% deoxycholate) containing protease inhibitors. The protein concentration was determined using the Bio-Rad protein assay reagent (Bio-Rad Laboratories, Hercules, CA, USA). Samples containing 30 μg of protein were boiled in sodium dodecyl sulfate (SDS) sample buffer at 95ºC for 5 minutes, separated by 10% SDS-polyacrylamide gel electrophoresis, and transferred to polyvinylidene difluoride membranes. Primary Abs (10 μg/mL) against NFATc1, c-Fos, TRAP, ERK 1/2, p-ERK, JNK, p-JNK, p38, p-p38 NF-κB (p65), IκBα, and β-actin were incubated overnight at 4ºC. Then the secondary HRP-conjugated Ab, which was goat anti-rabbit IgG or rabbit anti-goat IgG, was washed three times for 30 minutes. Reactive proteins were detected by enhanced chemiluminescence (Amersham Life Sciences, Arlington, IL, USA) using Fusion FX7 (Vilber Lourmat).
Cytochemical assessment of osteoclast formation (TRAP staining)
The BMMs were seeded at a density of 1.5 × 104 cells/well in a 96-well plate in triplicate for 3 days. The cells were cultured for 4 days with IL-1β (10 ng/mL) or atorvastatin (0.5 μM) in the presence of M-CSF (30 ng/mL) and RANKL (50 ng/mL). After 4 days, the cells were fixed with 3.7% formalin and permeabilized in 0.1% Triton X-100 for 10 minutes. The cells were stained with TRAP stain solution using a leukocyte acid phosphatase kit (Sigma-Aldrich) according to the manufacturer’s instructions. TRAP positive cells containing three or more nuclei were counted as multinuclear osteoclasts. Photographs were taken under a light microscope (Carl Zeiss, Thornwood, NY, USA).
Resorption pit assay
The formation of osteoclasts was evaluated by the resorption pit area as follows. The BMMs (2 × 104 cells/well) were seeded in a 96-well plate containing a dentine slice 5 mm in diameter (Immunodiagnostic Systems, Gaithersburg, MD, USA) with IL-1β (10 ng/mL) or atorvastatin (0.5 μM) in the presence of M-CSF (30 ng/mL) and RANKL (50 ng/mL) for 3 weeks. Cells on the dentine slices were rubbed with a cotton bud and stained with hematoxylin (Sigma-Aldrich) or TRAP. Quantification of the area of resorption was conducted using the IMT i-solution software (Samwoo Scientific Co., Seoul, Korea).
Statistical analysis
All data are expressed as a mean ± standard deviation of triplicate measurements. The mean values of each group were compared using Student t test or analysis of variance as appropriate, and p < 0.05 was considered statistically significance. Statistical analyses were performed using SPSS version 16.0 (SPSS Inc., Chicago, IL, USA).
RESULTS
Atorvastatin inhibits RANKL-mediated or IL-β-stimulated osteoclast differentiation
To determine the effects of atorvastatin on RANKL-mediated or IL-1β-stimulated osteoclastogenesis, we initially examined the formation of TRAP (+) multinuclear cells as an indicator of osteoclast differentiation, which satisfied most of the morphological criteria of osteoclasts. IL-β significantly increased the formation of TRAP (+) cells compared to the absence of this cytokine (48.2 ± 4.3 vs. 149.4 ± 7.5, respectively; p < 0.05). Atorvastatin significantly decreased RANKL-mediated or IL-1β-stimulated formation of TRAP (+) cells compared to the absence of atorvastatin (34.3 ± 4.5 vs. 47.3 ± 5.2, respectively; p < 0.05) (Fig. 1A).
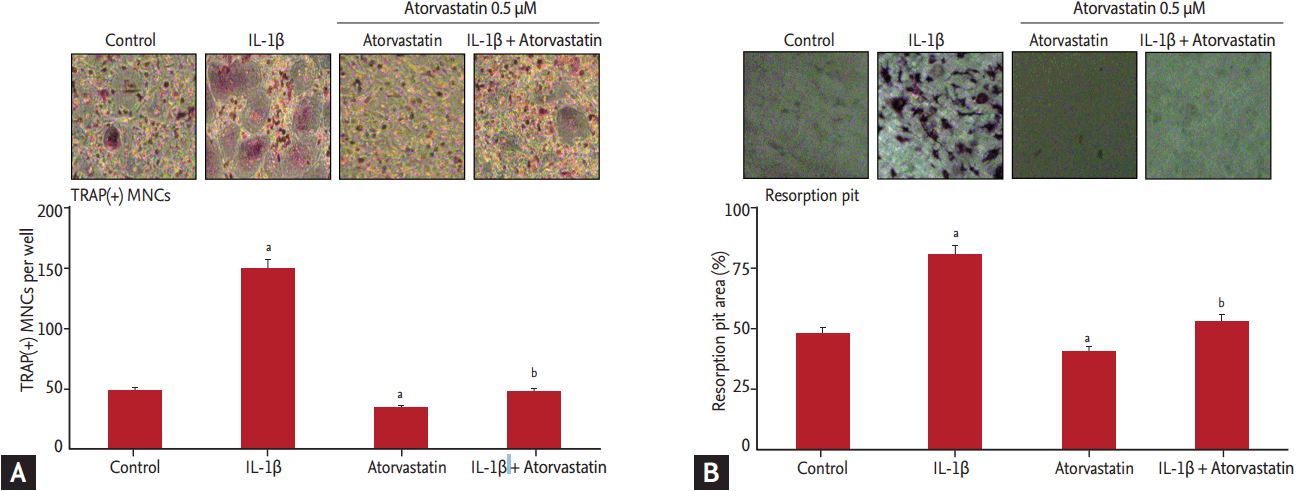
Inhibition of receptor activator of nuclear factor κB ligand (RANKL)-mediated, interleukin 1β (IL-1β)-induced tartrate-resistant acid phosphatase (TRAP) (+) cell formation by atorvastatin. (A) Osteoclast precursors were cultured with macrophage colony-stimulating factor (30 ng/mL) and RANKL (50 ng/mL) for 3 days in the presence or absence of IL-1β (10 ng/mL) or atorvastatin (0.5 µM). The cells were stained with TRAP solution. Atorvastatin significantly decreased RANKL-mediated or IL-1β stimulated formation of TRAP (+) cells compared to the absence of atorvastatin. (B) Atorvastatin (0.5 µM) also significantly decreased the RANKL-mediated or IL-1β-stimulated formation of resorption pits compared to the absence of atorvastatin. The results are presented as a mean ± standard deviation (n = 3). MNC, multinucleated cell. ap < 0.05 vs. no IL-1β and atorvastatin, bp < 0.05 vs. IL-1β without atorvastatin.
We also measured the resorption area as another indicator of osteoclast formation. IL-1β significantly increased the resorption area compared to the absence of IL-1β (48.3 ± 4.5 vs. 80.8 ± 3.2, respectively; p < 0.05). Atorvastatin also significantly decreased the RANKL-mediated or IL-1β stimulated formation of resorption pits compared to the absence of atorvastatin (40.9 ± 3.5 vs. 53.4 ± 2.5, respectively; p < 0.05) (Fig. 1B).
Atorvastatin inhibits RANKL-mediated or IL-β-stimulated survival of osteoclast precursors
To evaluate the effects of atorvastatin on the growth properties of the BMCs from the 5-week-old male ICR mice, we measured cell survival with M-CSF (30 ng/mL) and RANKL (100 ng/mL) in the presence or absence of atorvastatin (0.5 μM) or IL-1 (10 ng/mL) for 3 days as previously described. As shown in Fig. 2A, IL-1β significantly increased the survival of osteoclast precursor cells compared to the control without IL-1β (p < 0.05). Atorvastatin significantly inhibited the survival of osteoclast precursor cells compared to IL-1β (p < 0.05). However, there was no difference in the survival of osteoclast precursor cells between the control and cultures with atorvastatin. To determine the dose-dependent effects of atorvastatin on IL-1β-induced survival of osteoclast precursor cells, we added various doses of atorvastatin (0.5, 1, and 5 μM) to the osteoclast precursor cell cultures with IL-1β (10 ng/mL) for 3 days and performed a CCK-8 assay. The inhibitory effects of atorvastatin were significantly enhanced as the concentration of atorvastatin increased (Fig. 2B). These effects were also time dependent (data not shown).
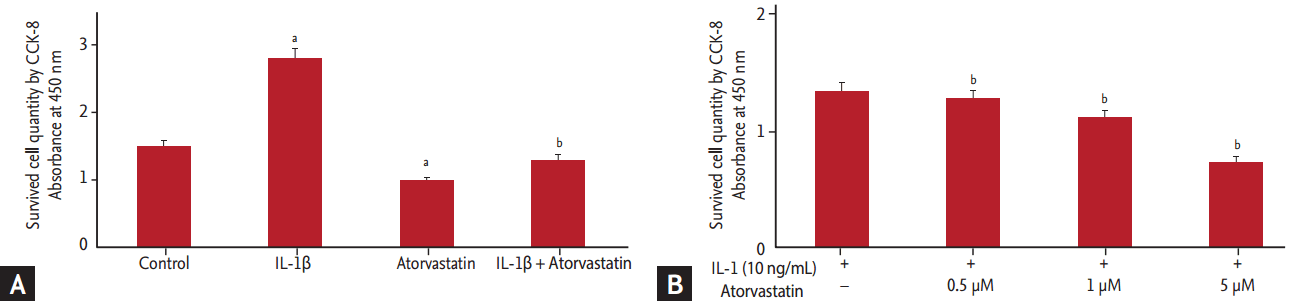
(A, B) Interleukin 1β (IL-1β) increases the tartrate-resistant acid phosphatase (TRAP) (+) cell formation and atorvastatin inhibits IL-1β-induced survival of osteoclast precursors dose-dependently. The survival of bone marrow cells is evaluated with a CCK-8 kit after culture for 3 days with/without IL-1β (10 ng/mL) and atorvastatin (0.5 µM). Atorvastatin significantly inhibited IL-1β stimulated proliferation of TRAP (+) multinucleated cells. The results are presented as a mean ± standard deviation (n = 3). Atorvastatin also inhibited IL-1β stimulated proliferation of TRAP (+) cell dose-dependently. ap < 0.05 vs. no IL-1β and atorvastatin, bp < 0.05 vs. IL-1β without atorvastatin.
Atorvastatin suppresses c-Fos and NFATc1 expression induced by RANKL or IL-1β
Osteoclast differentiation is regulated by the induction of various genes in response to RANKL and other osteotropic agents, including IL-1β and TNF-α. IL-1β is a very important inflammatory cytokine in RA and causes bone loss by increasing osteoclast formation. Both c-Fos and NFATc1 play essential roles in the differentiation of osteoclast precursors [10]. Therefore, we examined whether atorvastatin regulated the expression of c-Fos, NFATc1 messenger RNAs (m-RNAs), and proteins in response to RANKL or IL-1β. The densitometric value of reverse transcription (RT)-PCR normalized by the GAPDH intensity showed that atorvastatin significantly decreased the RANKL-mediated or IL-1β-stimulated expression of c-Fos and NFATc1 mRNA (Fig. 3A). Consistent with the results of the RT-PCR analyses, expression of c-Fos and NFATc1 protein levels increased in response to RANKL and IL-1β, and the expression of c-Fos and NFATc1 was significantly inhibited by atorvastatin (Fig. 3B). Thus, atorvastatin may inhibit osteoclast differentiation by inhibiting c-Fos and NFATc1 expressions in response to RANKL and IL-1β. Many molecules are implicated in cell-cell fusion, including E-cadherin [18], DC-STAMP [19], and Src family kinases [20]. Therefore, we examined whether atorvastatin regulated the expression of E-cadherin, DC-STAMP, and c-Src. The densitometric value of RT-PCR normalized by the GAPDH intensity showed that atorvastatin significantly decreased RANKL or IL-1β, as well as the expression of E-cadherin, DC-STAMP, and c-Src mRNA (Fig. 4).
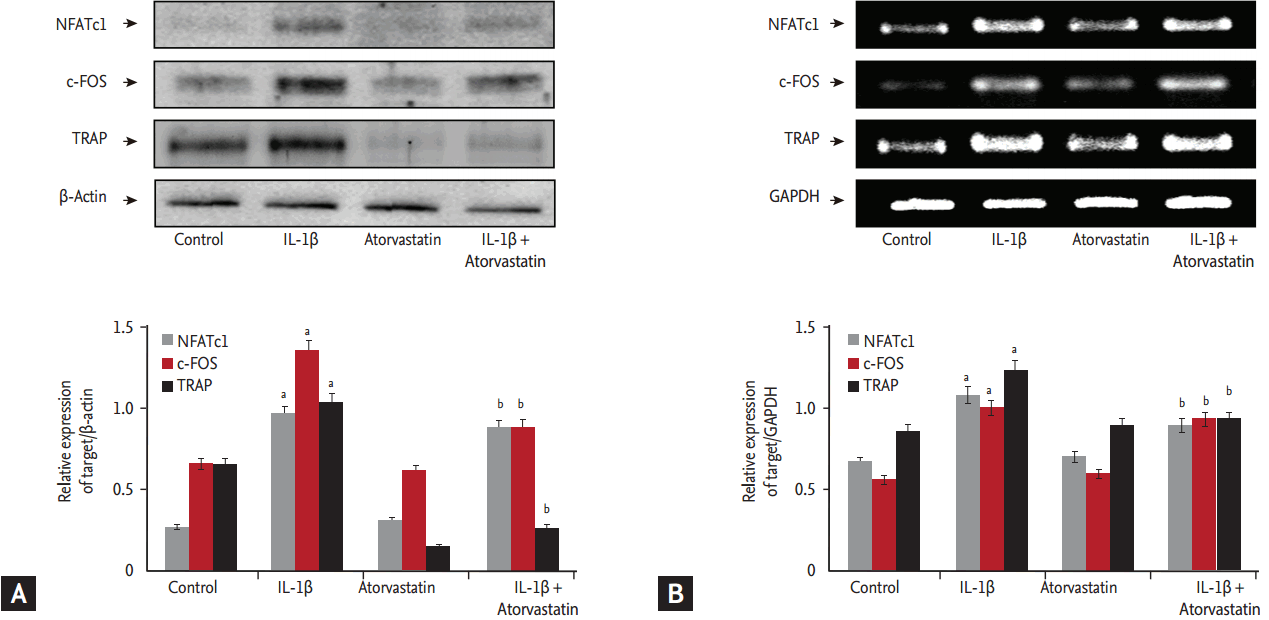
Effects of atorvastatin on interleukin 1β (IL-1β)-stimulated, receptor activator of nuclear factor-κB ligand-mediated nuclear factor of activated T-cells (NFATc1), c-Fos, and tartrate-resistant acid phosphatase (TRAP) activation. To evaluate the expression of NFATc1, c-Fos, and TRAP messenger RNA (mRNA) and protein, we performed immunoblotting and reverse transcription polymerase chain reaction. IL-1β enhanced the expression of NFATc1, c-Fos, and TRAP compared to the results without IL-1β. Atorvastatin (0.5 µM) inhibited the IL-1β stimulated expression of NFATc1, c-Fos, TRAP protein (A), and mRNA (B). The results are presented as a mean ± standard deviation (n = 3). GAPDH, glyceraldehyde 3-phosphate dehydrogenase. ap < 0.05 vs. no IL-1β and atorvastatin, bp < 0.05 vs. IL-1β without atorvastatin.
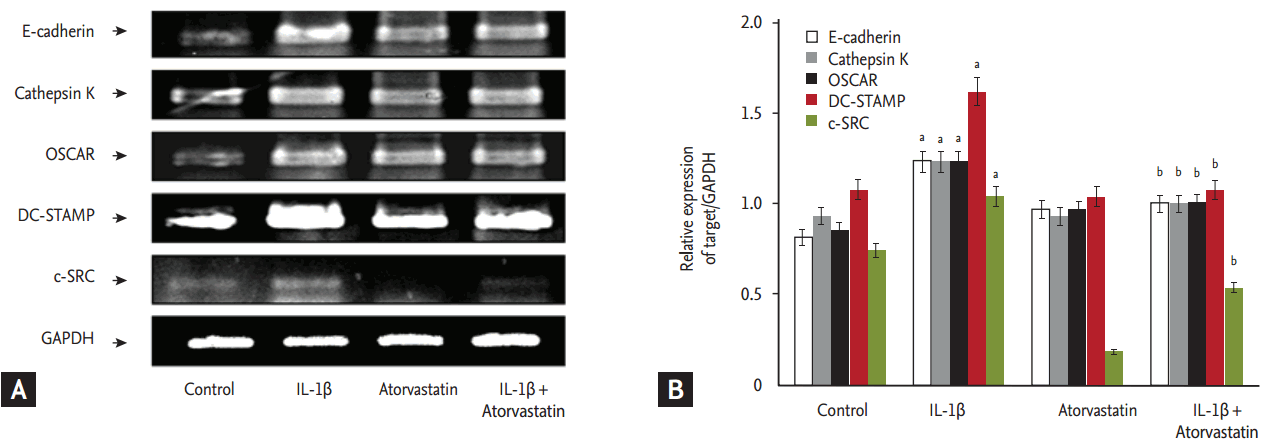
(A, B) Effects of atorvastatin on interleukin 1β (IL-1β)-stimulated, receptor activator of nuclear factor κB ligand-mediated dendritic cell-specific transmembrane protein (DC-STAMP), cathepsin K, osteoclast-associated receptor (OSCAR), DC-STAMP, and c-Src expression. IL-1β enhances the expression of E-cadherin, cathepsin K, OSCAR, DC-STAMP, and c-Src compared to the results without IL-1β. Atorvastatin (0.5 µM) inhibits the IL-1β-stimulated expression of E-cadherin, cathepsin K, OSCAR, DC-STAMP, and c-Src. The results are presented as a mean ± standard deviation (n = 3). GAPDH, glyceraldehyde 3-phosphate dehydrogenase. ap < 0.05 vs. no IL-1β and atorvastatin, bp < 0.05 vs. IL-1β without atorvastatin.
Atorvastatin suppresses MAPK signal pathways induced by RANKL or IL-1β
To determine the involvement of signal transduction and the mechanisms underlying the effect of atorvastatin on IL-1β-stimulated, RANKL-mediated osteoclast differentiation, we evaluated the activation of MAPKs in the BMCs. The densitometric values on immunoblots normalized according to β-actin intensity showed that IL-1β activated intracellular MAPKs, including ERK, p38, JNK (p < 0.05), and atorvastatin significantly inhibited RANKL-mediated or IL-1β-stimulated activation of intracellular MAPK, including ERK and JNK, except p38 (p < 0.05) (Fig. 5). These results indicated that atorvastatin inhibits RANKL-mediated or IL-1β-stimulated osteoclast differentiation and survival via intracellular MAPKs pathways.
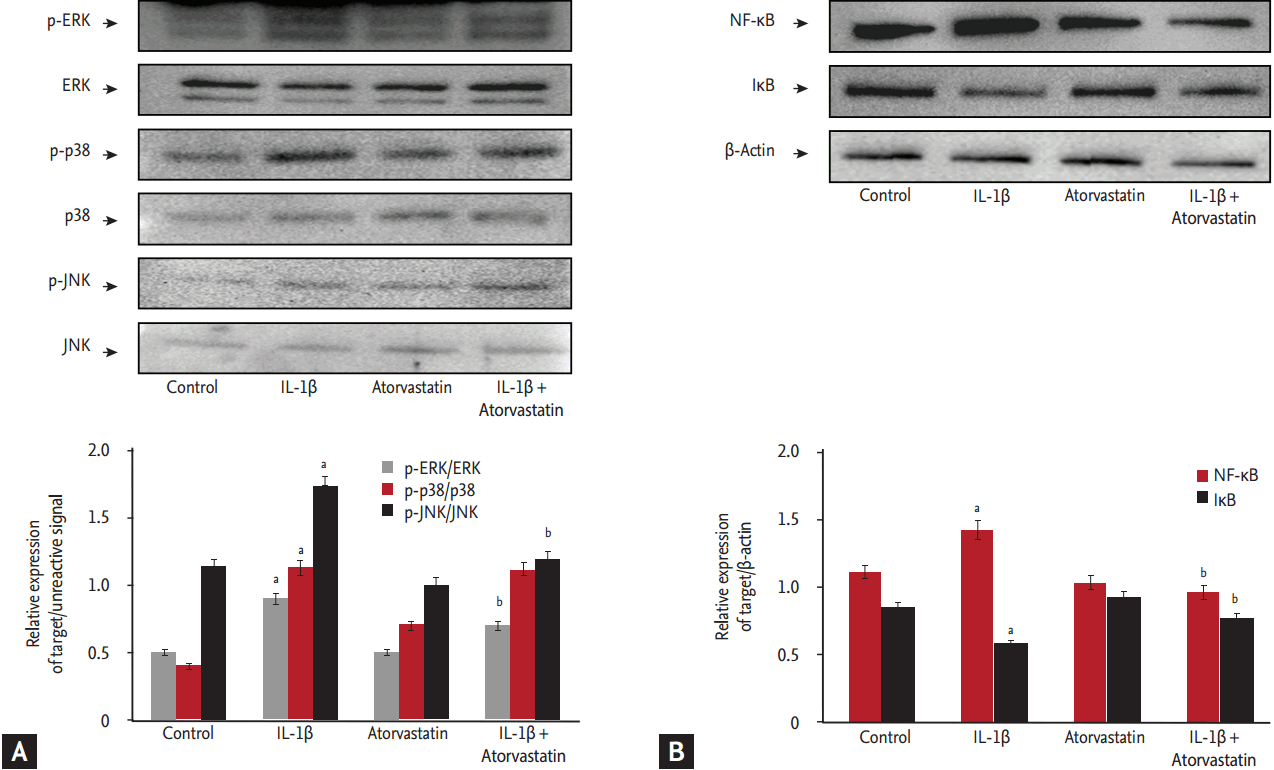
Effects of atorvastatin on interleukin 1β (IL-1β)-stimulated, receptor activator of nuclear factor-κB (NF-κB) ligand-mediated mitogen-activated protein kinase (MAPK) signal pathways and NF-κB activation. To evaluate the mechanism of atorvastatin on IL-1β-induced osteoclast differentiation, we evaluated the MAPK signaling pathway and NF-κB activation by immunoblotting. (A) IL-1β enhanced the phosphorylation of extracellular signal-regulated kinase (ERK), p38, and c-Jun N-terminal kinases (JNK), and atorvastatin inhibited the IL-1β-simulated activation of ERK, p38, and JNK. (B) IL-1β activated NF-κB and decreased cytoplasmic inhibitor of NF-κBα (IκB), and atorvastatin inhibited the IL-1β-stimulated activation of NF- κB. The results are presented as a mean ± standard deviation (n = 3). ap < 0.05 vs. no IL-1β and atorvastatin, bp < 0.05 vs. IL-1β without atorvastatin.
DISCUSSION
The modulation of bone formation and inflammation are considered among the various functions of statins [21-23]. Numerous clinical data have shown that statins modulated autoimmune disease activity or indeed modified vascular risk factors in rheumatic disease [24-26]. McCarey et al. [26] showed marked suppression with atorvastatin of acute-phase variables and a significant reduction in the swollen joint count in patients with RA presenting with active disease, despite existing disease-modifying antirheumatic drug therapy. There are many experimental studies on the effect of atorvastatin on osteoblasts, whereas there are only a few about the effect of atorvastatin on osteoclasts. In a recent study, atorvastatin markedly reduced RANKL expression in the fibroblast-like synoviocytes (FLSs) of patients with RA, and atorvastatin inhibited osteoclastogenesis in FLSs. Atorvastatin inhibits osteoclastogenesis and bone destruction in patients with RA [27]. A recent study suggested an inhabitant effect of atorvastatin on osteoclasts. Subsequently, no studies have reported on this effect. Therefore, our research team attempted to directly differentiate osteoclasts from a mouse and observe the effect.
The present study findings showed that atorvastatin inhibits IL-1β-stimulated, RANKL-mediated osteoclast differentiation in a dose-dependent manner by inhibiting activation of the ERK and p-JNK kinase as well as inhibiting c-Fos and NFATc1 expression. These findings suggest that atorvastatin has an important inhibitory role in bone loss by preventing osteoclast formation, and it may be useful as a new preventive agent for managing bone destruction in inflammatory diseases such as RA.
IL-1 is produced by many cell types relevant in rheumatoid synovitis and is readily detected in the rheumatoid joint, both in the synovial fluid (IL-1β) and within cells (IL-1α > IL-1β) [28]. IL-1β is critical in the pathogenesis of inflammatory synovitis and joint destruction in RA, as it induces the differentiation and activation of osteoclasts [29]. IL-1 can induce and enhance TRAF activation via heterodimeric IL-1 receptors. Thus, IL-1 differentiates and activates osteoclasts by engaging and activating TRAF6 via MAPKs, c-Fos, and NFATc1 signaling pathways [3,7]. However, IL-1 alone is unable to stimulate NFATc1 expression, but it can do so in the presence of a permissive level of RANKL [30]. RANKL plays a crucial role in IL-1 in terms of inducing the expression of NFATc1, which in turn cooperates with other transcriptional factors to simulate gene expression and thus osteoclastogenesis in osteoclast precursors. We found that atorvastatin inhibits osteoclast differentiation in a dose-dependent manner in IL-1β-stimulated, RANKL-mediated conditions. This suggests that atorvastatin may be a potent therapeutic compound for RA. However, further studies are required to investigate the overall effects and underlying mechanisms of atorvastatin on the pathophysiology of osteoclastogenesis in in vivo systems such as animal models of RA, including collagen-induced arthritis.
RANKL-RANK interaction activates many transcription factors, including NFATc1, a calcineurin and calcium-regulated transcription factor. RANKL induced the expression of c-Fos during early osteoclastogenesis. The binding of c-Fos to the NFATc1 promoter region is increased by RANKL, which induces NFATc1 gene expression [10,31]. The presence of NFATc1 in precursor cells prompts them to undergo osteoclastogenesis in the absence of RANKL [10]. In this study, to elucidate the mechanisms behind the effects of atorvastatin on IL-1β-induced, RANKL-mediated osteoclast differentiation, the expression of c-Fos and NFATc1 were examined. We found that the inhibition of osteoclast differentiation by atorvastatin coincided with the regulation of c-Fos and NFATc1. Furthermore, NFATc1 induced the expression of osteoclast-specific genes such as TRAP, cathepsin K, and OSCAR [9,32]. However, we found that atorvastatin synergistically reduced the expression of TRAP, cathepsin K, and OSCAR by inhibiting NFATc1.
The binding of RANKL to RANK also recruits TRAF-6 to activate all MAPK pathways, namely ERK, JNK, and p38 as well as phosphatidylinositol 3-kinase [33]. TRAF-6–/– mice are severely osteopetrotic either with abundant, dysfunctional osteoclasts [34] or without osteoclasts [35]. In the present study, to elucidate the mechanisms behind the effects of atorvastatin on IL-1β-induced, RANKL-mediated osteoclast differentiation, the expression of ERK, p38 MAPKs, and JNK were examined. We found that the inhibition of osteoclast differentiation by atorvastatin coincided with the regulation of ERK and p-JNK. However, further studies are required to define the precise mechanisms of these effects on osteoclast differentiation in the absence or presence of stimulating factors. Further investigations are also required to elucidate the mechanisms by which intracellular signaling factors are more specifically or directly involved in the effects of atorvastatin on osteoclast differentiation.
Much progress has been made in recent years for understanding osteoclast fusion and its regulation. DC-STAMP is a significant fusion regulator [19], and its expression is regulated by direct and indirect mechanisms [36]. Regarding the direct mechanism, RANKL/RANK downstream transcription factors, which are important for RANKL-mediated osteoclast formation, are critical for osteoclast fusion, and this includes c-Fos and NFATc1. The fact that atorvastatin down-regulated the fusion of osteoclast precursors led us to hypothesize that atorvastatin may control the expression of DC-STAMP. However, further investigations are required to define several important fusogenic genes, including the osteoclast stimulatory transmembrane protein [37], CD9 [38], ATP6v0d2 [39], and dose-dependent effect of atorvastatin on osteoclast fusion.
In conclusion, the findings presented herein demonstrated that atorvastatin inhibits IL-1β-stimulated, RANKLmediated osteoclast differentiation. This study also showed that atorvastatin inhibits the phosphorylation of MAPK pathways and the activation of c-Fos and NFATc1. These findings need to be validated in in vivo models prior to their extrapolation to the pathophysiological condition, and further evaluations are needed to define the exact mechanisms of atorvastatin’s intracellular signals on these effects. However, a better insight into the mechanisms of atorvastatin regarding osteoclast differentiation may provide a new therapeutic target for preventing articular bone and cartilage destruction in inflammatory joint diseases, including RA.
KEY MESSAGE
1. Atorvastatin has an important inhibitory role in bone loss by preventing osteoclast formation.
2. Atorvastatin may be useful as a new preventive agent for managing bone destruction in inflammatory diseases such as rheumatoid arthritis.
Notes
No potential conflict of interest relevant to this article was reported.
Acknowledgements
This study was supported by the Fund of Biomedical Research Institute, Chonbuk National University Hospital.