Blockade of thymic stromal lymphopoietin and CRTH2 attenuates airway inflammation in a murine model of allergic asthma
Article information
Abstract
Background/Aims
Thymic stromal lymphopoietin (TSLP) is an epithelial cell-derived cytokine that plays a key role in Th2-mediated inflammation, both directly by promoting the proliferation of naïve CD4 Th2 cells, and indirectly by activating dendritic cells (DCs). TSLP-activated DCs induce the expansion of chemoattractant receptor homologous molecule expressed on Th2 (CRTH2)+ CD4+ Th2 memory cells, which undergo a Th2 response and express prostaglandin D2 (PGD2) synthase. CRTH2, a PGD2 receptor, is a selective Th2-cell surface marker. We investigated the effects of an anti-TSLP antibody (Ab) and a CRTH2 antagonist, as well as their mechanisms of action, in a mouse model of acute asthma.
Methods
BALB/c mice were sensitized and challenged with ovalbumin. We then evaluated the effects of the administration of an anti-TSLP Ab either alone or together with a CRTH2 antagonist on cell counts, Th2 cytokine levels in bronchoalveolar fluid, and the levels of epithelium-derived cytokines such as TSLP, interleukin (IL) 33, and IL-25 in lung homogenates, as well as airway hyper-responsiveness (AHR).
Results
Anti-TSLP Ab and the CRTH2 antagonist significantly attenuated eosinophilic airway inflammation, AHR, and the expression of Th2 cytokines. The expression of GATA-3 and the levels of IL-33 and IL-25 in lung tissues were affected by the combined anti-TSLP and CRTH2 antagonist treatment.
Conclusions
These results suggest that the dual blockade of TSLP and CRTH2 may serve as an effective treatment target for eosinophilic asthma.
INTRODUCTION
Allergic asthma involves a chronic airway inflammation characterized by reversible airflow obstruction and airway hyper-responsiveness (AHR) [1]. Allergic airway inflammation is centrally driven by CD4+ Th2 cells, which produce Th2-type immune responses [2]. The airway epithelium is a key regulator of innate and Th2 immunity, and also plays a central role in asthma. A triad of cytokines, including interleukin (IL) 25, IL-33, and thymic stromal lymphopoietin (TSLP), are produced and released when airway epithelial cells are exposed to various stimuli [3]. These cytokines promote Th2-type immune responses by affecting various cell types involved in innate immune responses, such as innate type 2 lymphoid cells (ILC2s), dendritic cells (DC), and CD4+ T cells [4,5].
TSLP is an IL-7-like cytokine that interacts with the TSLP receptor (TSLPR), and it is known to be a master switch of allergic inflammation at the epithelial cell/DC interface [6]. TSLP induces the upregulation of OX40L expression on DCs. The interaction between OX40L and OX40 on naïve T cells causes polarization in Th2 cells, resulting in the release of Th2 cytokines, including IL-4, 5, 9, and 13 [7-9].
Moreover, the function of TSLP-activated DCs is not only to prime Th2-mediated immune responses, but also to sustain allergic inflammation by polarizing chemoattractant receptor homologous molecule expressed on Th2 (CRTH2)+ CD4+ Th2 effector memory cells [10]. A subpopulation of CD4+ T cells expresses CRTH2, a G protein-coupled receptor for prostaglandin D2 (PGD2). CRTH2+ CD4+ T cells are Th2-committed memory cells that produce IL-4, IL-5, and IL-13 upon T cell receptor triggering in humans [11,12]. CRTH2 is expressed on Th2 cells, eosinophils, and basophils, which have all been shown to contribute to the pathogenesis of human allergic diseases [12,13]. Fevipiprant, an anti-CRTH2 antagonist, has been developed as a new oral agent for the treatment of bronchial asthma. To date, the results of phase I and II clinical trials have shown efficacy in symptom control and a slight improvement in lung function [14,15]. However, the benefits of anti-CRTH2 antagonist have thus far been limited to atopic asthma, a subpopulation of bronchial asthma. Moreover, it has yet to show efficacy on severe asthma with phase III clinical trials [16].
A phase II clinical trial of tezepelumab, an anti-TSLP antagonist, has recently shown a successful reduction in acute exacerbation and type 2 airway inflammation. [17]. A phase III clinical trial evaluating the efficacy of anti-TSLP antagonist is currently ongoing. In previous clinical trials, both anti-TSLP antagonist and CRTH2 antagonist have been found to be effective for suppressing type 2 inflammation; however, the clinical benefits and positioning of these agents have yet to be clarified. An unmet need for the use of these biologics targeting bronchial asthma still persists, because effective biomarkers or clinical strategies for both drug initiation and response evaluation have yet to be established. Laboratory pre-clinical experiments as well as clinical trials might be useful for improving current asthma treatment strategies.
The objective of this study was to investigate the effect and benefit of the dual inhibition of TSLP and CRTH2 on epithelium-derived cytokines, Th2 responses, and AHR in a mouse model of asthma.
METHODS
Animals
Female BALB/c mice, seven to eight weeks of age, were obtained from KOATECH (Pyeongtaek, Korea) and maintained in specific pathogen-free conditions. These BALB/c mice were divided into four groups (n = 5 to 8 per group): control (CON), ovalbumin (OVA) challenge, OVA plus anti-TSLP antibody (Ab) (OVA + anti-TSLP), and OVA plus anti-TSLP Ab and CRTH2 antagonist (OVA + anti-TSLP & CRTH2 antagonist) treatment. All animal care and experimental procedures were in accordance with guidelines and were approved by the Animal Subjects Committees of the Catholic University of Korea (CUMS-2014-0184-02).
Sensitization, antigen challenge, and drug treatment
Mice were sensitized by a subcutaneous injection of 25 μg of OVA (grade V, Sigma-Aldrich, St. Louis, MO, USA) adsorbed in 1 mg of aluminum hydroxide (Aldrich, Milwaukee, WI, USA) in phosphate-buffered saline (PBS) on days 0 and 7. They were challenged four times by intranasal administration of OVA (20 μg/50 μL in PBS), beginning on day 21. On each day of OVA challenge, mice were given either 1mg/kg of anti-TSLP Ab (MAB555, R&D Systems, Minneapolis, MN, USA) intranasally or 7 mg/kg CRTH2 antagonist (TM30089, ChemieTek, Indianapolis, IN, USA) orally 2 hours before the challenge. Anti-TSLP Ab and TM30089 were dissolved in PBS. The control group was treated in the same way, with PBS without OVA. Animals were sacrificed 24 hours after the last OVA challenge.
Measurement of airway AHR
AHR was assessed by measuring changes in the airway resistance (cmH2O·s/mL) of mice after exposure to methacholine (Mch, 6.25 to 50 mg/mL, Sigma-Aldrich) in PBS using the Flexivent system (SCIREQ, Montreal, QC, Canada). Prior to sacrificing the mice, they were anesthetized with a mixture of Zoletil (30 mg/kg, Virbac Laboratories, Carros, France) and Rompun (10 mg/kg, Bayer, Leverkusen, Germany), tracheostomized (stainless steel cannula, 18 G), and mechanically ventilated (160 breaths/ min, tidal volume of 10 mL/kg). Then, Mch aerosol was administered for 10 seconds, after which airway resistance was continuously monitored and recorded.
Obtaining samples from BAL fluid
Immediately following blood collection, the trachea was exposed, and bronchoalveolar lavage (BAL) fluid was collected by cannulating the upper part of the trachea and lavaging twice with 1 mL PBS. BAL fluid was centrifuged at 2,000 rpm for 5 minutes at 4℃. After centrifugation, lavage supernatants were removed, pellets were resuspended in 300 μL PBS, and total viable cell numbers were counted by trypan blue exclusion using a haemacytometer. Cells were centrifuged at 750 ×g for 5 minutes using a cytospin, then stained with Diff-Quik (Sysmax, Tokyo, Japan). Differential inflammatory cell counts were obtained by counting 400 leukocytes on randomly selected areas of slides using light microscopy. Supernatants were stored at –70℃.
Lung tissue histology
Left lung tissues were fixed in 4% paraformaldehyde, embedded in paraffin, and sectioned at 3 μm. Tissue sections were subjected to haematoxylin and eosin (H&E) and periodic acid Schiff (PAS) staining. The magnification used was 200 times magnification. In order to quantify goblet cell hyperplasia, the pathological changes were evaluated according to the method described by Padrid et al. [18]. For each mice, two to four airways from each lung lobe were evaluated, and the percentage of goblet cells in the epithelial lining cells were scored separately by the two researchers as follows: no goblet cells = 0, < 25% = 1, 25% to 50% = 2, 51% to 75% = 3, and > 75% = 4. The grading data are presented in histograms.
Enzyme-linked immunosorbent assay
Cytokines were measured by enzyme-linked immunosorbent assay (ELISA). The protocol followed was according to the manufacturer’s instructions (IL-4, -5, -10, -13, -25, -33, interferon γ [IFN-γ], TSLP [R&D Systems, Minneapolis, MN, USA], transforming growth factor β [TGFβ], total immunoglobulin [Ig] E, and OVA-specific IgG1 [eBiosciences, Leicestershire, UK]). The concentrations of IL-4, -5, -10, -13, IFN-γ, and TGFβ were measured in the BAL fluid, and the levels of IL-25, -33, and TSLP were measured in the lung lysate. Blood was allowed to clot at room temperature for 2 hours, then centrifuged at 3,000 rpm for 15 minutes. Aliquots of serum were analyzed for total IgE and OVA-specific IgG1 [19]. For OVAspecific IgG1, the microplate was coated with purified rat anti-mouse IgG1, then treated with mouse sera followed by biotinylated OVA, which is generated with a peroxidase conjugated goat anti-biotin antibody, which was used as secondary antibody. The reactions were read using an ELISA plate reader at 450 nm.
RNA extraction, cDNA synthesis, and real-time polymerase chain reaction
Total cellular RNA was isolated from lung tissues using Trizol reagent. The total RNA (1 μg) was directly reverse-transcribed using a PrimeScript First Strand cDNA Synthesis Kit (RR037A, TaKaRa, Shiga, Japan) according to the manufacturer’s protocol. The reverse transcription reaction was incubated at 37℃ for 15 minutes, inactivated at 85℃ for 5 seconds, and then cooled at 4℃. Quantitative real-time polymerase chain reaction (qRT-PCR) was performed using a SYBR FAST qPCR Kit Master Mix (2X) Universal (KR0389, KAPA biosystems, Boston, MA, USA) with forward and reverse primers for GATA-binding protein-3 (GATA-3) and GAPDH. The primer sequences used were as follows: GATA-3 sense, 5- TCATTAAGCCCAAGCGAAGG-3; GATA-3 anti-sense, and 5- GTCCCCATTGGCATTCCTC-3. PCRs were carried out in a real time PCR Machine (CFX96 Touch) (Bio-Rad, Irvine, CA, USA). The samples were incubated at 95℃ for 3 minutes, followed by 40 cycles at 95℃ for 5 seconds and then at 60℃ for 30 seconds. Each sample was amplified in duplicate. The fold change of test gene mRNA was expressed as 2-ΔΔCt (ΔCt = the difference in threshold cycles for the test gene and GAPDH, ΔΔCt = the difference of ΔCt between stimulated and non-stimulated control).
Statistical analysis
The data were subjected to one-way analysis of variance (ANOVA), followed by Dunnett’s multiple range test using GraphPad Prism version 5.00 for windows (GraphPad Software, San Diego, CA, USA). All data are expressed as mean ± SD, and in all cases, p < 0.05 was considered to be statistically significant.
RESULTS
AHR
The OVA group showed significantly increased respiratory system resistance (Rrs; cmH2O·s/mL) to Mch at all doses as compared to control. Rrs values in response to mchmethacholine (25 to 50 mg/mL) decreased significantly after the anti-TSLP and CRTH2 antagonist treatment (p < 0.001) (Fig. 1).
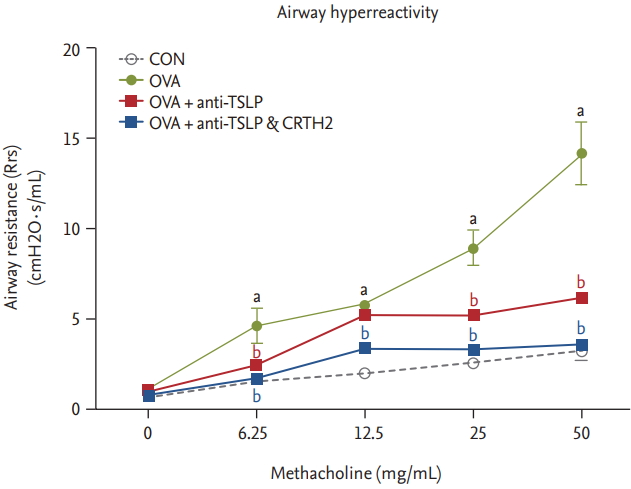
Effect of thymic stromal lymphopoietin (TSLP) and chemoattractant receptor homologous molecule expressed on Th2 (CRTH2) antagonist on airway hyperresponsiveness (AHR) to methacholine. AHR was measured 24 hours after the final ovalbumin (OVA) challenge using a Flexivent system in which mice were exposed to increasing concentrations of methacholine (6.25 to 50 mg/mL). Values are expressed as mean ± SEM (n = 5 to 8/group). CON, control. a p < 0.001 compared with the CON group, b p < 0.001 compared with the OVA group.
Inflammatory cell influx
The mean total inflammatory cell, eosinophil, and neutrophil counts in BAL fluid were significantly elevated in the OVA group versus the control group (Fig. 2). Significant decreases were observed in total inflammatory cell and eosinophil counts in the anti-TSLP treatment group (76.4 ± 24.3 × 104/mL and 36.9 ± 10.9 × 104/mL, respectively) as compared to those in the OVA group (144.3 ± 40.8 × 104/mL and 74.0 ± 33.1 × 104/mL, respectively; and neutrophil: 34.5 ± 2.0 × 104/mL) (p < 0.001 and p < 0.01, respectively). Significant decreases in the total inflammatory cell, eosinophil, and neutrophil counts were observed in the anti-TSLP plus CRTH2 antagonist treatment group (58.9 ± 27.8 × 104/mL, 28.3 ± 17.6 × 104/ mL and 5.7 ± 6.0 × 104/mL, respectively) as compared to those in the OVA group (p < 0.001, p < 0.001, and p < 0.05, respectively).
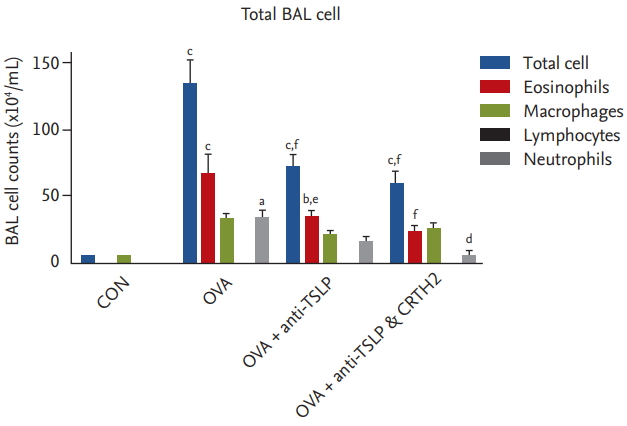
Effect of thymic stromal lymphopoietin (TSLP) and chemoattractant receptor homologous molecule expressed on Th2 (CRTH2) antagonist on total and differential cell counts in bronchoalveolar lavage (BAL) fluid. Mice were sacrificed 24 hours after the final ovalbumin (OVA) challenge, and BAL cells were isolated. Values are expressed as mean ± SEM (n = 5 to 8/group). CON, control. a p < 0.05, b p < 0.01, and c p < 0.001 compared with the CON group, d p < 0.05, e p < 0.01, and f p < 0.001 compared with the OVA group.
Histopathological studies showed increased peribronchial eosinophilic infiltration in the OVA-treated group as compared to the control group. The anti-TSLP plus CRTH2 antagonist treatment decreased the accumulation of inflammatory cells (Fig. 3A). Airway epithelial and goblet cell hyperplasia with mucus overproduction was detected in the OVA-treated group stained with Alcian blue/periodic acid–Schiff. The anti-TSLP plus CRTH2 antagonist treatment reduced epithelial and goblet cell hyperplasia as well as mucus secretion (Fig. 3B and 3C) as compared to the OVA group.
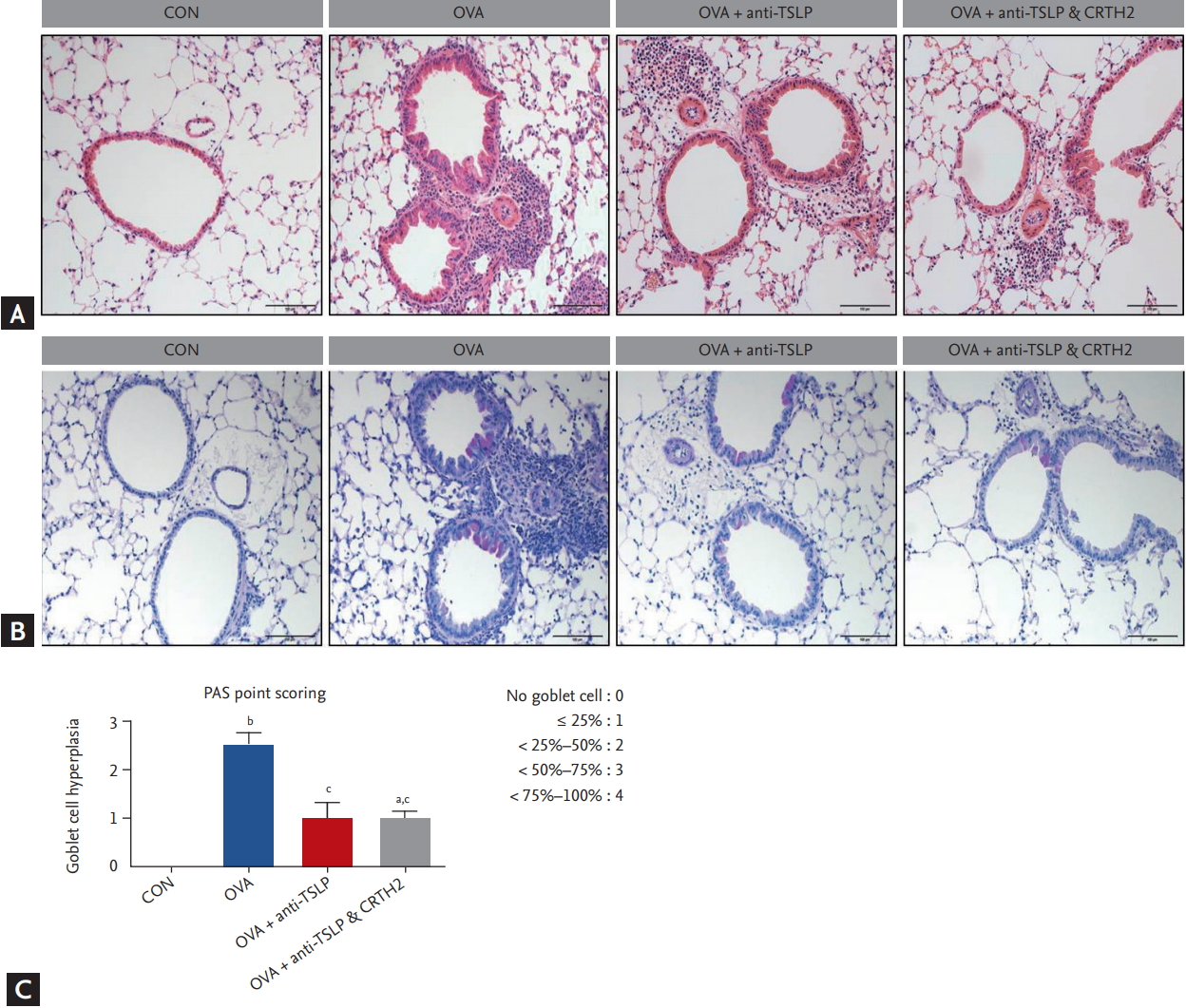
Effect of thymic stromal lymphopoietin (TSLP) and chemoattractant receptor homologous molecule expressed on Th2 (CRTH2) antagonist on lung histopathology and goblet cell hyperplasia. Representative photomicrographs of (A) H&E and (B) periodic acid Schiff (PAS) stained lung sections (×200) from mice of the treatment groups are shown. (C) Quantification of the PAS-stained area by point scoring. Values are expressed as mean ± SEM (n = 5 to 8/group). CON, control; OVA, ovalbumin. a p < 0.05 and b p < 0.001 compared with the CON group, c p < 0.01 compared with the OVA group.
Cytokine levels in lung homogenate
The expression of TSLP and IL-33 in lung tissue homogenates was significantly upregulated in the OVA group as compared to the control group. Treatment with anti-TSLP effectively inhibited TSLP and IL-33 in lung tissues; the addition of the CRTH2 antagonist decreased the IL-33 level as compared to that in the OVA group (p < 0.001) (Fig. 4A and 4B).
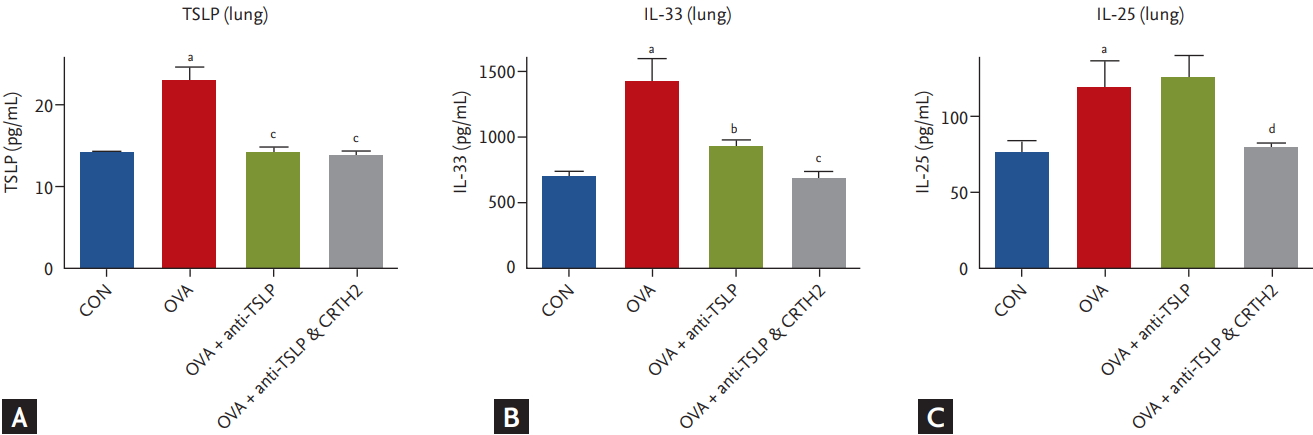
Effect of thymic stromal lymphopoietin (TSLP) and chemoattractant receptor homologous molecule expressed on Th2 (CRTH2) antagonist on TSLP signaling related cytokine levels in lung tissues. Concentrations of (A) TSLP, (B) interleukin 33 (IL-33), and (C) IL-25 in lung tissue were measured by enzyme-linked immunosorbent assay (ELISA) assay. Values are expressed as mean ± SEM (n = 5 to 8/group). CON, control; OVA, ovalbumin. a p < 0.001 compared with the CON group, b p < 0.01, and c p < 0.001 compared with the OVA group, d p < 0.05 compared with the OVA + anti-TSLP group.
IL-25 expression was also strongly upregulated in the OVA group as compared to the negative control. The addition of the CRTH2 antagonist to anti-TSLP resulted in decreased IL-25 levels as compared to those with anti-TSLP alone treatment (p < 0.05) (Fig. 4C).
Cytokine levels in BAL fluid
As shown in Fig. 5, mice immunized with OVA showed significantly increased IL-4, IL-5, and IL-13 levels as compared to those in the control group. Mice pretreated with anti-TSLP only and together with the CRTH2 antagonist showed markedly inhibited expression of IL-5 and IL-13. No difference was observed in IL-4 level in the treatment group as compared to that in the OVA group (Fig. 5A-5C).
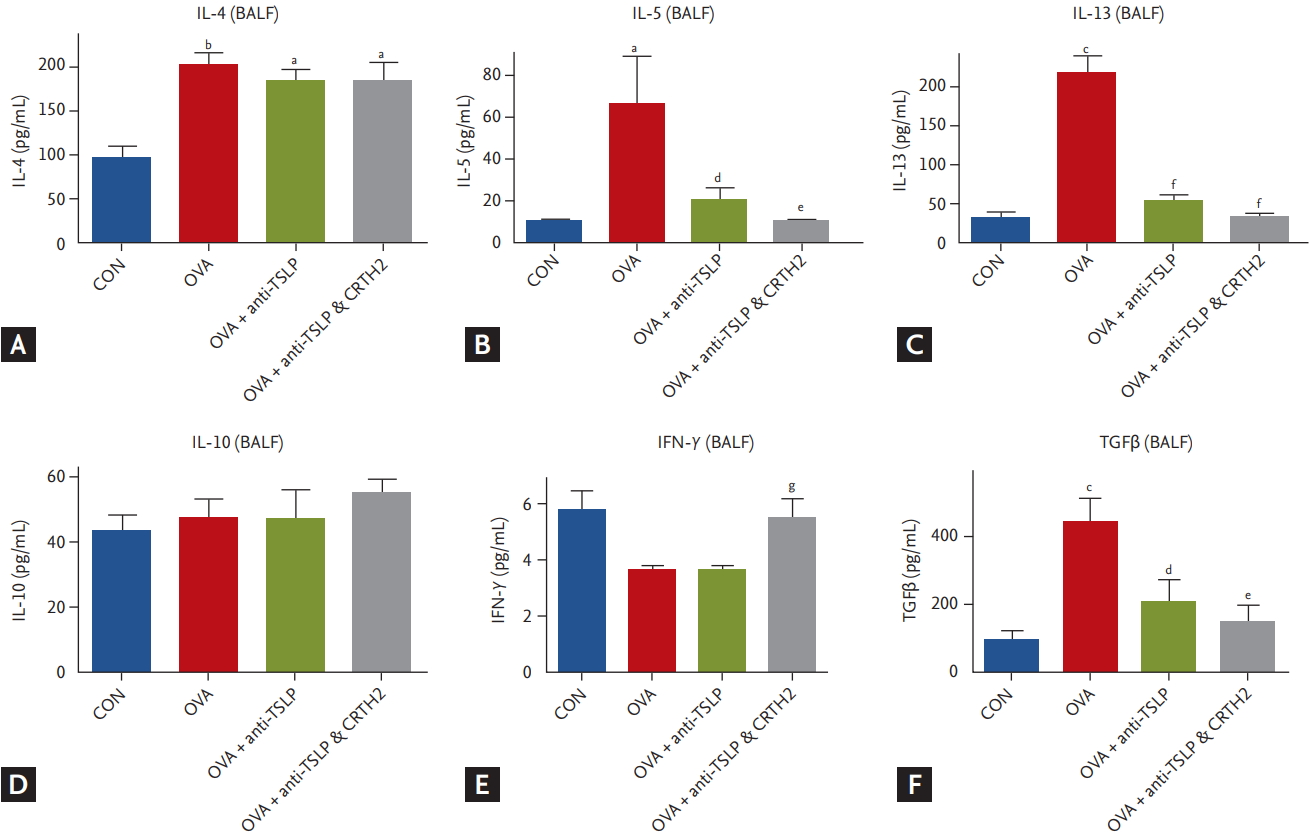
Effect of thymic stromal lymphopoietin (TSLP) and chemoattractant receptor homologous molecule expressed on Th2 (CRTH2) antagonist on asthma related cytokine levels in bronchoalveolar lavage fluid (BALF). (A, B, C) Concentrations of Th2 cytokines (interleukin 4 [IL-4], IL-5, and IL-13) in BALF were measured by enzyme-linked immunosorbent assay (ELISA) assay. Values are expressed as mean ± SEM (n = 5 to 8/group). (D, E, F) Concentrations of anti-inflammatory cytokine, IL-10, Th1 cytokines (IFN-γ) and transforming growth factor β (TGFβ) in BALF were measured by ELISA assay. Values are expressed as mean ± SEM (n = 5 to 8/group). CON, control; OVA, ovalbumin. a p < 0.05, b p < 0.01, and c p < 0.001 compared with the CON group, d p < 0.05, e p < 0.01, and f p < 0.001 compared with the OVA group, g p < 0.05 compared with the OVA + anti-TSLP group.
IL-10 expression did not differ between the treatment and OVA groups (Fig. 5D). IFN-γ expression was considerably lower in the OVA and anti-TSLP-treated groups than in the control group. Anti-TSLP plus CRTH2 antagonist treatment significantly increased the levels of IFN-γ as compared to those in the anti-TSLP only treatment group (p < 0.01) (Fig. 5E).
TGFβ1 expression was increased significantly in the OVA group as compared to the control group. Blockade with anti-TSLP and the CRTH2 antagonist had an inhibitory effect on TGFβ1 production (p < 0.05, p < 0.01) (Fig. 5F).
Serum levels of total IgE and OVA-specific IgG1
Total IgE and OVA-specific IgG1 levels were significantly higher in the OVA-treated group than in the control group. Treatment with anti-TSLP only or together with the CRTH2 antagonist reduced the levels of total IgE and OVA-specific IgG1 (p < 0.05) (Fig. 6) as compared to those in the OVA group.
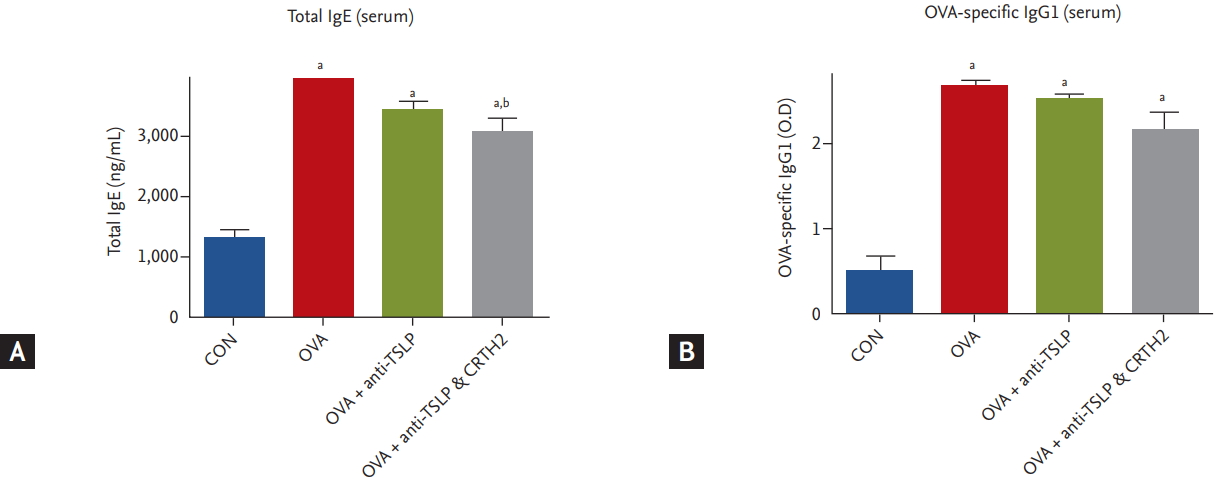
Effect of thymic stromal lymphopoietin (TSLP) and chemoattractant receptor homologous molecule expressed on Th2 (CRTH2) antagonist on immunoglobulin in serum. Concentrations of (A) total interleukin E (IgE) and (B) ovalbumin (OVA)-specific IgG1 were measured in serum by enzyme-linked immunosorbent assay (ELISA) assay. Values are expressed as mean ± SEM (n = 5 to 8/group). CON, control; O.D., optical density. a p < 0.001 compared with the CON group, b p < 0.05 compared with the OVA group.
GATA-3 and T-bet mRNA expression levels in lung homogenate
We analyzed the expression levels of GATA-3 and T-bet in the Th1/Th2 signaling pathway. GATA-3 and T-bet are transcription factors expressed during differentiation from naïve T cells to Th1 and Th2 cells. T-bet is essential for Th1 development while GATA-3 is essential for Th2 development [20]. GATA-3 mRNA expression was increased significantly in the OVA group as compared to in the control group. The anti-TSLP plus CRTH2 antagonist treatment significantly decreased GATA-3 mRNA expression (p < 0.05) (Fig. 7A) as compared to the OVA group. The ratio of GATA-3/T-bet also decreased as compared to that in the OVA group (Fig. 7B).
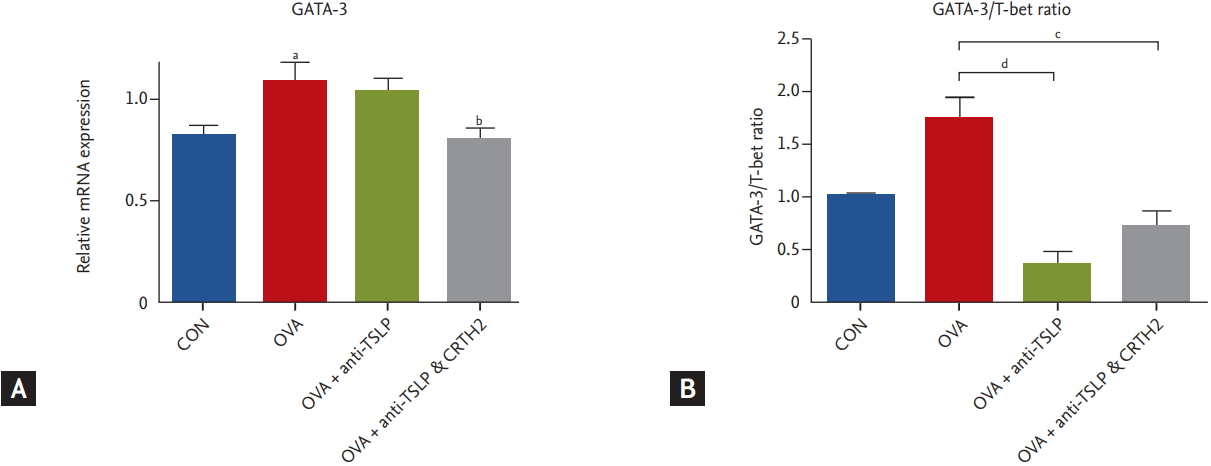
Effect of thymic stromal lymphopoietin (TSLP) and chemoattractant receptor homologous molecule expressed on Th2 (CRTH2) antagonist on key transcriptional factors for Th2 cell. (A) The relative quantitative mRNA levels of GATA-3 and (B) the ratio of GATA-3/T-bet in the lung homogenate were detected by real-time polymerase chain reaction. The levels of gene expression were normalized to the expression of β-actin. The comparative CT method (ΔΔCT) was used for quantification of gene expression. CON, control; OVA, ovalbumin. a p < 0.001 compared with the CON group, b p < 0.05 compared with the OVA group, c p < 0.01, d p < 0.001 compared with the OVA group.
DISCUSSION
The dual blockade of TSLP and CRTH2 effectively suppressed eosinophilic airway inflammation, AHR, goblet cell hyperplasia, and the expression of Th2 cytokines in an OVA-induced acute asthma model. The decreased level of GATA-3 transcription factor shown in this study indicated that the dual blockade of TSLP and CRTH2 may have affected the type 2 airway inflammation, consisting of ILC2s and Th2 mediated response in asthmatic mice. Additional interesting findings included the levels of epithelial-derived cytokines, IL-25 and IL-33, following treatment with anti-TSLP and the CRTH2 antagonist. While IL-33 was reduced in mice treated with either anti-TSLP alone or both, IL-25 and GATA-3 were reduced only in mice that received both anti-TSLP and the CRTH2 antagonist.
TSLP is an epithelial cell-derived cytokine expressed in the skin, gut, lungs, and thymus. TSLP plays a key role in Th2-mediated inflammation both directly, by promoting the proliferation of naïve CD4 Th2 cells, and indirectly, by activating DCs [8,10,21]. In addition to these types of adaptive immunity, TSLP is involved in innate immunity stimulated by nonatopic eosinophilic airway inflammation mediated by ILC2s. TSLP acts as one of the “alarmins” reflecting airway epithelial injury by viruses, fungi, and pollutants, as well as allergens [22]. TSLP challenge or overexpression of TSLP in the lungs of transgenic mice induced asthmatic responses [23]. By contrast, mice lacking the TSLPR show a reduced allergic response in the airways [24]. Adding anti-TSLPR antibody or an anti-TSLP monoclonal antibody has been shown to alleviate Th2-mediated airway inflammation in animal models of asthma [25,26].
CRTH2 is a G protein-coupled receptor for PGD2, a major mediator released from activated mast cells. CRTH2 mediates the mast cell-dependent activation of both Th2 lymphocytes and eosinophils, while PGD2 stimulates Th2 cytokines, AHR, and the production of Th2 cytokines and IgE. Allergic responses are enhanced through the action on CRTH2 [11-13].
We showed that the blockade of TSLP decreased AHR, eosinophilic airway inflammation, and goblet cell hyperplasia. In addition, the levels of Th2 cytokines, such as IL-5 and IL-13, in BAL fluid decreased significantly after anti-TSLP antibody treatment. No changes were observed in the levels of IL-10 or IFN-γ, but the TGFβ1 levels in BAL fluid decreased significantly after the anti-TSLP antibody treatment.
Adding the CRTH2 inhibitor to anti-TSLP antibody treatment led to a significant increase in IFN-γ levels as compared to treatment of anti-TSLP alone. It is well known that IL-10 is released from regulatory T cells and suppresses Th2-related allergic reactions [27]. Further, IFN-γ is a well-known Th1 cytokine. The increased expression of IFN-γ would be associated with decreased levels of Th2 cytokines, such as IL-4, IL-5, and IL-13. In order to assess changes in the Th1/Th2 ratio, we analyzed the ratio of IL-4 and IFN-γ levels in BAL fluid and showed that they decrease compared to the OVA group after anti-TSLP alone and both TSLP/CRTH2 antagonists (data not shown). The results supported the anti- Th2 effects of TSLP and CRTH2 blockades.
The profibrotic growth factor TGFβ1 is expressed by eosinophils and plays an important role in airway inflammation and the promotion of airway remodeling, as expressed by subepithelial fibrosis and the transformation of fibroblasts into myofibroblasts [28,29]. Our data showed that pretreatment with anti-TSLP antibody alone or together with CRTH2 antagonists led to significant decreases in the number of airway eosinophils and TGFβ1 levels in mice.
Th1/Th2 homeostasis is mediated by T-bet and GATA- 3, which are key transcription factors during the differentiation of naïve T cell into Th1 and Th2 cells, respectively. One study revealed that the Th2 response in the airway depends on a shift in T-bet/GATA-3 homeostasis in the lung toward greater GATA-3 activity [30,31]. As expected, in our study, GATA-3 was overexpressed in the OVA group. Anti TSLP/CRTH2 antagonist administration decreased GATA-3 expression as compared to that of OVA-administered mice. This effect was the same in the ratio of GATA-3/T-bet shown in Fig. 7B. This result is interesting because the expression of GATA-3 represents the first step in the Th-cell effector response to polarizing signals, and surface CRTH2 has been used as a molecular identifier for the Th2 subsets, downstream of GATA-3 [32]. We assumed that GATA-3 suppression could be through ILC2s, because TSLP could induce GATA-3 expression in human ILC2s through signal transducer and the activator of transcription 5 [33]; further, GATA-3 is known to regulate the development, maintenance, and function of ILC2s [34]. However, these direct effects on ILC2s should be evaluated in further studies. This remains as one of the main limitations of our study.
OVA induced an increase in all three epithelial cell markers (TSLP, IL-33, and IL-25), in lung homogenate as compared to the control group in our study. Our results showed that anti-TSLP significantly reduced not only TSLP, but also IL-25 and IL-33 levels, which is an interesting finding. Several reports have described interactions among these epithelial-derived cytokines. For example, neutralizing IL-25 reduced IL-33 and TSLP expression, while an IL-33 antagonist decreased the expression of IL-25 and TSLP in a house dust mite-induced bronchial asthma model [35,36]. Moreover, TSLPR knockout mice showed decreased expression of IL-25 after a respiratory virus infection [37]. Moreover, the suppression of IL-25 expression by CRTH2 plus TSLP antagonist could be due to the difference between the cells producing IL-25 and IL-33. IL-25 is produced in innate effector eosinophils and basophils [38], and IL-33 is a tissue-derived cytokine from endothelial, epithelial, and fibroblast-like cells [39]. The suppression of both CRTH2 and TSLP may have affected the level of innate effector eosinophils more than endothelial cells. However, as the mechanisms of action remained unclear, the effects of anti-CRTH2 on epithelial-derived cytokines will be evaluated further in future research.
Bronchial asthma is a heterogenous disease, and researchers have categorized patients with bronchial asthma by underlying molecular mechanisms called endotype. The Th2 high and Th2 low endotypes are primarily discussed, according to the characteristics of airway inflammation. Th2 high endotype is mediated by eosinophilic airway inflammation and Th2 low endotype by neutrophils or minimal inflammatory cells (paucigranulocytic) [40]. Type 2 high asthma is developed by both adaptive and innate immune pathways, mainly stimulating Th2 cells and ILC2s individually. Recently, asthmatic patients mediated by non-atopic stimuli such as virus, microbes, and pollutants have been shown to be different from traditional atopic, allergen-induced patients. Current biologics targeting eosinophilic airway inflammation in atopic patients include IL-5/IL-5 receptor antagonist and monoclonal antibody to IL-4/IL-13 [40]. However, targeting eosinophilic asthma without atopy is challenging, because agents directly targeting ILC2s have yet to be developed. The results of our study suggest that the dual blockade of anti-TSLP and CRTH2 suppressed the expression of GATA-3, a strong activator of ILC2s in addition to IL-25, which is involved in both innate immunity and Th2 cells. Our results support the hypothesis that the addition of anti-CRTH2 antagonist to the anti-TSLP antibody may intensify the inhibitory effects of eosinophilic airway inflammation with additional effects on innate immune response in difficult-to-treat asthma cases.
There are some limitations to our study. First, we used a mouse model of acute asthma, and CRTH2 in human Th2 cells may differ from that in mice. However, previous studies have reported that mouse CRTH2 bore 77% shared identity with its human homologue, and the use of CRTH2 antagonist in a cockroach egg antigen-induced mouse asthma model made effectively decreased Th2 related gene expression [36,41]. Secondly, since the effect of the anti-CRTH2 antagonist group was not evaluated, the additional effects of dual blockade with anti- CRTH2 inhibitor and anti-TSLP antibody could not be clearly addressed. Since the CRTH2 receptor is involved in the induction of the migration and activation of Th2 lymphocytes, eosinophils, and basophils, comparing the effect of CRTH2 antagonist with dual blockade would be meaningful. However, in previous reports, CRTH2 antagonist in several animal asthma models has shown decreased eosinophilic airway inflammation, AHR in terms of Th2 cell mediated airway inflammation only [42,43]. The decreased effect of GATA-3 expression and IL-25 would be novel in our experiments. The difference between the anti-CRTH2 inhibitor alone and anti-CRTH2/TSLP antibody should be evaluated further in future research. Third, the effects of anti TSLP and CRTH2 inhibitor on Th1/Th2 signaling pathway should be further evaluated by mouse splenocytes or lymph nodes. The analysis by the differences of Th1/ Th2 cytokines and GATA-3 gene expressions in lung tissues could be insufficient to explain immune response during antigen sensitization.
In conclusion, the blockade of TSLP and TSLP/CRTH2 effectively alleviated eosinophilic airway inflammation, goblet cell hyperplasia, and AHR, and also modulated type 2 response including GATA-3 expression in a mouse model of acute asthma. The dual blockade of TSLP and CRTH2 may represent a useful approach to treating eosinophilic asthma.
KEY MESSAGE
1. The dual blockade of anti-thymic stromal lymphopoietin (TSLP) and chemoattractant receptor homologous molecule expressed on Th2 (CRTH2) inhibitor successfully inhibited eosinophilic airway inflammation in an ovalbumin-induced acute asthma mice model.
2. The anti-asthmatic effect of anti-TSLP and CRTH2 inhibitor was induced by the suppression of type 2 immune response including GATA-3 expression.
3. The use of CRTH2 inhibitor in addition to anti-TSLP antibody may provide a useful treatment approach to treating eosinophilic asthma.
Notes
No potential conflict of interest relevant to this article was reported.